
Leaf Extract of Sedum kamtschaticum Fisch. & Mey. Ameliorates Cognitive Dysfunction in Trimethyltin-treated Rats










This is an open access article distributed under the terms of the Creative Commons Attribution Non-Commercial License (http://creativecommons.org/licenses/by-nc/3.0/) which permits unrestricted non-commercial use, distribution, and reproduction in any medium, provided the original work is properly cited.
Abstract
Alzheimer’s disease (AD) is a major neurodegenerative disorder and major type of dementia with progressive cognitive deficits, including multifactorial disorders. The present study examined the therapeutic effect of Sedum kamtschaticum leaf extract (SKE) on cognitive dysfunction disorder induced in rats through trimethyltin (TMT) administration.
In the preliminary screening experiment, SKE was selected as an acetylcholinesterase (AChE) inhibitor. Rats in the negative control and SKE groups were intraperitoneally injected with TMT (8 ㎎/㎏ body weight) dissolved in 0.9% saline and normal rats were injected with 0.9% saline alone. Doses of SKE (100, and 300 ㎎/㎏) were orally administered to the rats for 14 days. Normal and control rats were treated with saline for the same period. We performed a behavioral test using a Morris water maze and found that the escape and latency time periods of the SKE300 rats were shorter than those of the control rats. SKE therefore ameliorated cognitive dysfunction in this animal model. Immunohistochemical assay of biomarkers in the hippocampal cells revealed that the cell levels of phosphorylated cAMP response element binding protein (p-CREB) and AChE in the CA3 region of the control group were lower than those in the normal group. However, p-CREB and AChE reactive hippocampal cells in SKE300 group were significantly increased compared to those of the control. The hippocampal cells of brain-derived neurotrophic factor (BDNF) and protein kinase C (PKC) in control rats tended to be lower than the normal group, while the cells in the SKE groups tended to be higher than the control group.
These results suggest that SKE ameliorates cognitive disorders through the neuroprotective effects related to p-CREB and AChE in the hippocampal cells.
Keywords:
Sedum kamtschaticum Fisch. & Mey, Acetylcholinesterase, Cognition, Phosphorylated cAMP Response Element Binding Protein, TrimethyltinINTRODUCTION
Alzheimer’s disease (AD) has been known as the major type of dementia with progressive cognitive deficits as primary symptoms (Law et al., 2001). Several factors such as genetic predisposition, reduced synthesis of the excitatory neurotransmitter ACh, extracellular deposition of amyloid beta (Aβ) in the brain, abnormalities in tau protein leading to the formation of neurofibrillary tangles resulting in disintegration of microtubules, and oxidative stress and inflammatory cascades mediated by primed glial cells have been proposed as causes of AD (Singhal et al., 2014).
AD is a complex multifactorial disorder and has been linked to a deficiency in the brain neurotransmitter acetylcholine (ACh) (Tabet, 2006; Galimberti and Scarpini, 2016).
ACh, a neuromodulator, exerts cholinergic signaling effects on cellular and synaptic properties of neurons in several brain areas and influences personal behavior (Picciotto et al., 2012). Loss of the cholinergic markers, acetylcholinesterase (AChE) and choline acetyltransferase (ChAT), is the most consistent neurotransmitter alteration found in the brains of patients with AD. A progressive cognitive deterioration was documented in transgenic mice overexpressing human AChE in brain neurons which support the role of neocortical ACh in spatial memory (Talesa, 2001). Acetylcholinesterase inhibitors (AChEIs) could be introduced for the treatment of AD (Tabet, 2006). Hippocampal AChE activity is only reduced in mild cognitive impairment and early AD (Rinne et al., 2003), where AChEIs are used for mild to moderate treatment of AD (Galimberti and Scarpini, 2016).
In recently, materials from plant resources to improve memory were screened by our team (Park et al., 2018), and were reported from the results of mice experiments (Park et al., 2019; Kim et al., 2020).
Sedum kamtschaticum has been used as traditional medicine in North Asia to treat inflammatory disorders and its in vivo anti-inflammatory activity has been recently reported in ear edema-induced mice and paw edema-induced rats (Kim et al., 2004). The antimicrobial activity of S. kamtschaticum leaf and root extracts against Gram-negative and -positive bacteria were also reported (Kang et al., 2011). However, neither the in vitro nor in vivo effects of S. kamtschaticum on memory or cognition improvement have been investigated to date.
In the present study, S. kamtschaticum leave extract (SKE), as a potent inhibitor of AChE activity, was selected from the preliminary screening experiment and its memory improvement potential was evaluated in rats with cognitive disorder induced by trimethyltin (TMT) treatment. Rat behavior was examined through Morris water maze (MWM) test. The hippocampal cells were treated with the antibodies of phosphorylated cAMP response element binding protein (p-CREB), AChE, brain-derived neurotrophic factor (BDNF), and protein kinase C (PKC), and were assessed through immunohistochemical (IHC) staining.
MATERIALS AND METHODS
1. Plant extract preparation
Leaves of S. kamtschaticum Fisch. & Mey. were collected on 24 July 2018 at Eumseong, in Korea, which was identified by comparison with a specimen of the medicinal plant in the National Institute of Horticultural and Herbal Science (voucher no. MPS002557). The medicinal compound was extracted with 70% ethanol at 85℃ using a refluxing apparatus, and the solvent in the extract was eliminated by evaporation and freeze-drying. This leaf extract was then used for in vitro and in vivo studies.
2. Reagents
5,5'-dithio-bis-[2-nitrobenzoic acid], sodium phosphate, acetylthiocholine iodide, butyrylthiocholine iodide, AChE enzyme, BuChE enzyme, Tacrine, trimethyltin (TMT), sodium nitrate, heparin, 4% paraformaldehyde, diaminobenzidine-HCl, and hydrogen peroxide were purchased from Sigma-Aldrich Co. (St. Louis, MO, USA). Sodium pentobarbital was purchased from Hanlim Pharm Co., Ltd. (Seoul, Korea), phosphate buffer was done from Biosesang (Seongnam, Korea), and bovine serum albumin was done from Millipore (Billerica, MA, USA). Avidin-biotin complex kit was used as Vectastain ABC kit (Vector Laboratories, Burlingame, CA, USA). Antibodies of p-CREB and PKC were supplied from Cell Signaling (Denver, MA, USA) and Abcam (Cambridge, MA, USA), respectively. Antibodies of AChE and BDNF were provided by Santacruz Biotechnology Inc. (Dallas, Texas, USA).
3. Measurement of acetylcholinesterase (AChE) and butyrylcholinesterase (BuChE) inhibition effect
In vitro AChE and BuChE inhibitory activity of the SKE was evaluated according to the method of Kim and Chung (Kim and Chung, 2011).
Each sample (75 ㎕) of SKE, with final concentrations of 25, 50, 100, and 200 ㎍/㎖, was dispensed into a 96-well plate before the addition of 130 ㎕ of sodium phosphate buffer (100 mM, pH 8.0), 5 ㎕ of 10 mM 5,5'-dithio-bis-[2-nitrobenzoic acid] (DTNB) reagent, and 1 ㎕ of 75 mM acetylthiocholine iodide (or butyrylthiocholine iodide) substrate.
After 10 min, 2.5 ㎕ of AChE (or BuChE) enzyme (0.5 U/㎕ in buffer) was added to the mixtures and allowed to react for 1 hour, before absorbance was measured at 410 ㎚ with a microplate reader (Bio-Tek Instruments, Winooski, VT, USA). Tacrine (0.1 ㎍/㎖) was used as positive control and analyzed using the same procedure.
4. Animals, experimental design and drug treatment
Seven-week-old male Sprague-Dawley rats weighing 250 g - 280 g were purchased from the Samtaco Co. (Osan, Korea) and randomly divided into four groups (n = 8 - 9): normal, control, SKE100, and SKE300. For the decision of SKE administration doses, the literature reported by Kehr et al (2012), that the p.o. treatment of Ginko biloba extract (EGb 761®) of 100 ㎎/㎏ and 300 ㎎/㎏ for 14 days have caused a marked elevation in extracelluar dopamine levels of rat, was referred.
The animals were allowed to acclimatize for at least 7 days prior to the experiment and housed in individual cages under light-controlled conditions (12/12 hour, light/dark cycle) at room temperature (23℃) with ad libitum access to food and water. All the experiments were approved by the Kyung Hee University institutional animal care and use committee [KHUAP(SE)-18-073, 05/30/2018].
This experimental protocol was also permitted by an Institutional Review Committee for the use of Human or Animal Subjects to ensure that procedures are in compliance with at least the Declaration of Helsinki for human subjects, the National Institutes of Health Guide for Care and Use of Laboratory Animals (Publication No. 85 - 23, revised 1985), the England Animals Scientific Procedures Act 1986, or the European Communities Council Directive of 24 November 1986 (86/609/EEC).
Rats in the negative control and SKE groups were injected intraperitoneally (i.p.) with TMT (8 ㎎/㎏, single dose) dissolved in 0.9% saline on the first day and the normal rats were injected with 0.9% saline, following which all rats were returned to their original cages. Two doses of the SKE (100 ㎎/㎏ and 300 ㎎/㎏) were orally administered to rats in the SKE groups for 14 days. Normal rats and control rats were treated with saline for the same period.
5. Behavioral test using the Morris water maze
The swimming pool of the MWM comprised a circular water tank of 200 ㎝ in diameter and 35 ㎝ deep. It was filled to a depth of 21 ㎝ with water at 23 ± 2℃. A platform, 15 ㎝ in diameter and 20 ㎝ in height, was placed inside the tank with its top surface at 1.5 ㎝ below the surface of the water. The pool was surrounded by many cues that were external to the maze. A CCD camera was equipped with a personal computer for behavioral analysis. Each rat received four daily trials. For 4 consecutive days, the rats were tested with three acquisition tests.
They also received retention tests on the 5th day. For the acquisition test, the rat was allowed to search for the hidden platform for 180 s, following which the latency to escape onto the platform was recorded. The animals were trained to find the platform that was in a fixed position during the 4 days of the acquisition tests, and then for the retention test (on the 5th day), they received a 1 min probe trial in which the platform was removed from the pool. The inter-trial interval time was 1 min.
The performance of the test animals in each water maze trial was assessed by a personal computer for behavioral analysis Smart program (3.0 version, PanLab Co., Barcelona, Spain).
6. Immunohistochemistry
The rats were anesthetized with sodium pentobarbital (80 ㎎/㎏, i.p.) and transcardially perfused with a saline solution containing 0.5% sodium nitrate and heparin (10 U/㎖) and then fixed with 4% paraformaldehyde (PFA) in 0.1 M phosphate buffer (PB).
Each brain was dissected from the skull, post-fixed overnight in buffered 4% paraformaldehyde at 4℃, stored in a 30% sucrose solution at 4℃ until it sank, and frozen-sectioned on a sliding microtome into 30 ㎛ thick coronal sections. All sections were processed for immunohistochemical staining as described previously (Ye et al., 2014).
The brain sections were rinsed in PBS and then incubated overnight at room temperature with the primary antibody. The following day, the brain sections were rinsed with PBS and 0.5% bovine serum albumin (BSA), incubated with the appropriate biotinylated secondary antibody, and processed with an avidin-biotin complex kit. The bound anti-serum was visualized by incubation with 0.05% diaminobenzidine-HCl (DAB) and 0.003% hydrogen peroxide in 0.1 M PB. The DAB reaction was terminated by rinsing the tissues with 0.1 M PB. The primary antibodies that were used were directed against p-CREB (1 : 500), AChE (1 : 500), BDNF (1 : 500), and PKC (1 : 500). The labeled tissue sections were then mounted on gelatin-coated slides and analyzed with a bright-field microscope (DP2-BSW imaging system, Olympus Life Science, San Diego, CA, USA).
7. Statistical analysis
The data are presented as the means ± standard error of the mean (SEM). Statistical analyses in vitro assay were assessed using Duncan’s Multiple Range Test (DMRT) of SAS program (version 7.10, SAS Institute Inc., Cary, NC, USA), at p < 0.05. Statistical comparisons among different groups in vivo experiment were analyzed using One-way ANOVA, followed by the Tukey’s post-hoc test.
RESULTS AND DISCUSSION
1. In vitro inhibitory activity on AChE and BuChE
The fact that ACh coordinates the response of neuronal networks in many brain areas makes cholinergic modulation an essential mechanism underlying complex behavior (Picciotto et al., 2012). The use of AChE inhibitors elevates the concentration of ACh in treated tissues and has the ability to enhance cognitive performance (Chen et al., 2018).
BuChE activity progressively increases in patients with Alzheimer's disease (AD), while AChE activity remains unchanged or declines. Both enzymes therefore represent legitimate therapeutic targets for ameliorating the cholinergic deficit considered to be responsible for the declines in cognitive, behavioral and global functioning characteristic of AD (Greig et al., 2002).
This experiment was conducted to evaluate the inhibitory effect of SKE on AChE and BuChE activity in vitro. The results of the assay revealed that the SKE (50, 100, and 200 ㎍/㎖) inhibited AChE activity (20.0% - 54.3%) through dose-dependent manner. In vitro inhibitory effect of SKE (100, and 200 ㎍/㎖) inhibited BuChE activity (4.2% - 18.9%) through dose-dependent manner. However, the results for SKE on AChE and BuChE were lower than those (69.1% and 79.5% for 0.1 ㎍/㎖) for Tacrine used as a positive control drug (Fig. 1).
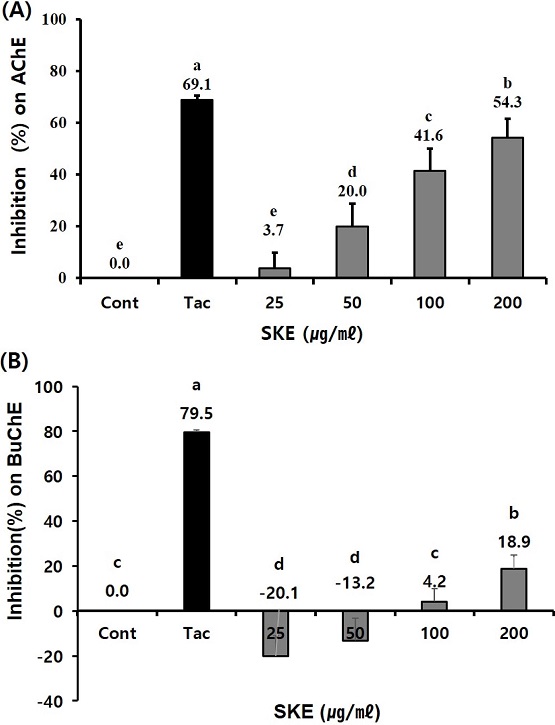
Effect of Sedum kamtschaticum leaf extract (SKE) treatment on (A) acetylcholinesterase (AChE) and (B) butyrylcholinesterase (BuChE) activity. SKE, with final concentrations of 25, 50, 100, and 200 ㎍/㎖, was reacted into a 96-well plate of 100 mM sodium phosphate buffer (pH 8.0), 10 mM DTNB reagent, 75 mM acetylthiocholine iodide (or butyrylthiocholine iodide) and AChE (or BuChE) enzyme. Absorbances of the reactants were measured at 410 ㎚. Tacrine (0.1 μg/㎖) was used as positive control. Means ± SD from triple test data is presented (n = 3 - 4). Means with different letters are significantly different at p < 0.05 by Duncan’s Multiple Range Test (DMRT).
2. Effect of SKE on memory-dysfunction behavior
Trimethyltin (TMT) is an intermediate by-product in the production of other more commonly used tin compounds and thus still constitutes an occupational hazard for some groups (Koczyk, 1996). TMT has been detected in human urine samples from individuals with no known acute exposure suggesting the possibility for environmental exposure to TMT and/or methylation of other tin species in vivo (Jenkins and Barone, 2004).
TMT produces a dose-dependent degeneration of neurons in the limbic system, particularly the hippocampus, amygdale and entorhinal cortex (Park, 2011), and causes a selective loss of pyramidal neurons in the hippocampal Cornu Ammonis (CA) 3 region and spatial memory impairment in rats (Koda et al., 2008). Necrosis of hippocampal pyramidal cells and granule cells by TMT, is associated with the disruption of normal behavioral patterns, hippocampal physiological activity and neurochemical markers of endogeneous hippocampal neurotransmitters. TMT intoxication attenuates hippocampal-dependent behavior in the Morris water maze. Therefore, TMT intoxication can be used to induce chronic neuronal degeneration related with cognitive impairment and is useful in AD study (Ye et al., 2020).
The MWM test is a reliable tool to measure spatial learning in rodents and is useful to investigate the correlation with hippocampal synaptic plasticity and N-methyl-D-aspartate (NMDA) receptor function (Ye et al., 2014). The beneficial effects of natural materials to treat cognitive dysfunctions such as AD have been previously evaluated with behavioral tests, including the MWM test (Sadeghi et al, 2018; Saleem et al., 2019).
To evaluate the spatial learning ability, all rats were subjected to MWM tests for 5 days after the 16th experiment day. On the 2nd and 3rd day of the MWM test, the escape time increased in the control rats (p < 0.01) compared to that of the normal rats, but significantly reduced in the SKE 300-administered rats (p < 0.05) compared to that of the control rats [p < 0.05, Fig. 2(A)].
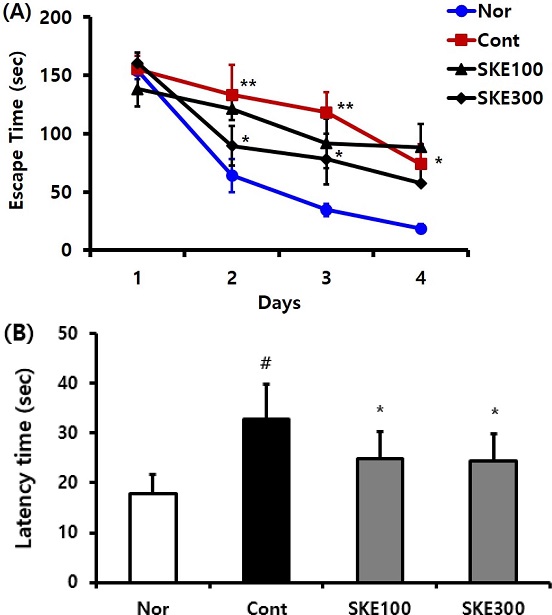
Effect of Sedum kamtschaticum leaf extract (SKE) administration on (A) escape time and (B) latency time in the Morris water maze test. Rats were randomly divided into four groups. Nor; normal, 0.9% saline, Cont; negative control, 0.9% saline + TMT 8 ㎎/㎏), SKE100; 100 ㎎/㎏ of Sedum kamtschaticum leaf extract in 0.9% saline + TMT 8 ㎎/㎏), and SKE300; 300 ㎎/㎏ of Sedum kamtschaticum leaf extract in 0.9% saline + TMT 8 ㎎/㎏), (n = 8 - 14). All materials were administered daily to rats for 21 days. #p < 0.05 versus normal. Comparisons among different groups were analyzed using One-way ANOVA, followed by the Tukey’s post-hoc test.
In the present study, the increasing of escape time in the control rats, was observed and the same results were examined in the control rats of the other studies. The result that the treatment of TMT plus SKE 300 ㎎/㎏ for 2 weeks reduced the escape time compared with the control group, was also examined in the other literatures of TMT plus herbal formular PM012 or TMT plus bean-phosphatidy serine (Jung et al., 2013; Ye et al., 2020).
The latency time which was increased in the control rats compared with the normal rats was reduced in the TMT plus SKE-treated rats. The TMT plus SKE300-treated rats showed the tendency of decreasing the latency time, compared to that of the control rats [p < 0.05, Fig. 2(B)]. The attenuation of latency time in SKE groups compared with that of the control group in the present work was also confirmed in the decreasing tendency of latency time of TMT and bean-phosphatidy serine-treated rats through Morris water maze test (Ye et al., 2020).
Therefore, the escape time and the latency time of the SKE-treated rats, especially the SKE300 group, were shorter than those of the control rats, and the results from the behavior test indicated that SKE ameliorates cognitive dysfunction induced in the studied animal model.
3. Effect of SKE on the hippocampal p-CREB immunoreactive cell level
Loss of neurons in the entorhinal cortex, hippocampus, and frontal, parietal and temporal cortices has been documented in patients with AD (West et al., 1994). Neurons in layer II of the entorhinal cortex and hippocampal CA1 neurons are particularly vulnerable, which might be related to the expression of genes that either promote or prevent neuronal death. Genetic and pharmacological studies have provided strong evidence that the CREB signaling pathway is crucial for learning and memory across species (Shim et al., 2012). TMT intoxication exerts its toxic effects on pyramidal neurones, especially in CA4/CA3 region and CA1 subfields (Koczyk, 1996). The death of neurons in the AD brain is characteristic of a form of programmed cell death called apoptosis (Mattson, 2004).
The CREB signaling pathway plays a critical role in memory formation and participates in the BDNF pathway that regulates cognitive function in the hippocampus. Phosphorylation of CREB is necessary for CRE-driven induction of gene transcription (Plátenı́k et al, 2000; Zhang et al, 2018).
Immunohistochemistry staining was conducted on the hippocampal p-CREB cell level in the control and SKE-administered groups. The p-CREB-ir cell levels in the CA1 region of the normal, the control, SKE100, and SKE300 groups were 51.8 ± 2.8, 39.4 ± 2.3, 50.6 ± 5.1, and 49.6 ± 3.0, respectively (p < 0.05). The p-CREB-ir cell levels in the CA3 region of the normal, the control, SKE100, and SKE300 groups were 61.8 ± 4.4, 35.1 ± 3.5, 46.4 ± 3.7, and 51.4 ± 3.4, respectively (p < 0.05).
The increasing tendencies in p-CREB immuno-reactive cell of SKE groups compared with those of control in CA1 and CA3 region were also examined by Shim et al. (2012) who suggested that Krill-derived phosphatidylserine significantly increased the CREB positive neurons in the hippocampal CA1 area as compared with that of the vehicle group.
Based on these results, it can be concluded that the SKE300 group significantly restored p-CREB-ir neurons in the CA1 and CA3 regions which were decreased in the control group (p < 0.05, Fig. 3), in contrast, the SKE100 group exhibited an increasing tendency.
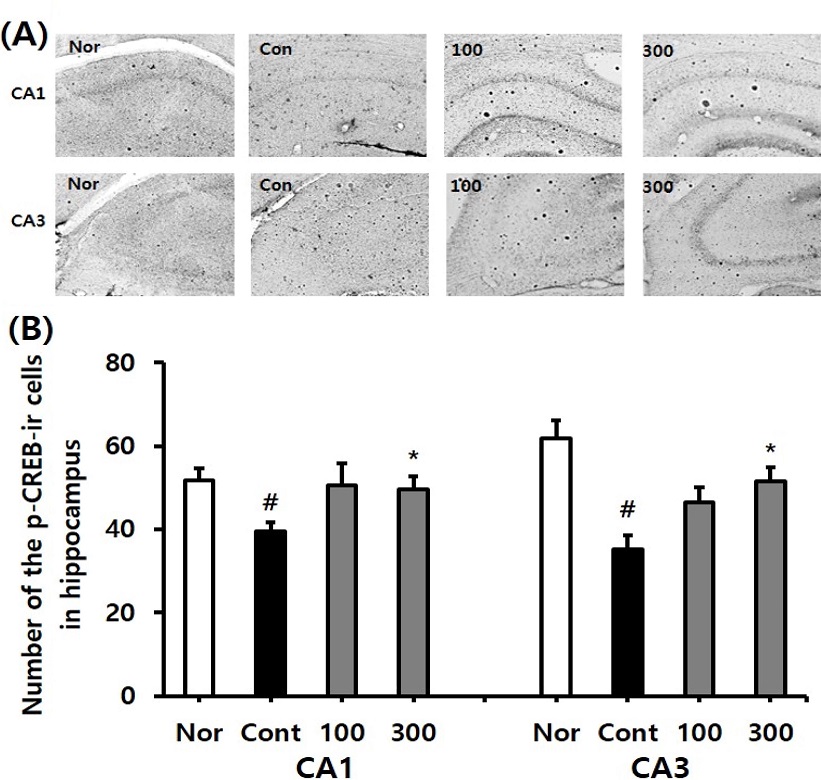
Effect of different concentrations of Sedum kamtschaticum leaf extract (SKE) administration on the p-CREB immunoreactive cell levels after trimethyltin treatment by immunohistochemistry. The representative photographs and the number of the p-CREB immunostained cells are indicated in (A) and (B). Rats were randomly divided into four groups. Nor; normal, 0.9% saline days, Cont; negative control, 0.9% saline + trimethyltin 8 ㎎/㎏), SKE100; 100 ㎎/㎏ of Sedum kamtschaticum leaf extract in 0.9% saline + trimethyltin 8 ㎎/㎏), SKE300; 300 ㎎/㎏ of Sedum kamtschaticum leaf extract in 0.9% saline + trimethyltin 8 ㎎/㎏) (n = 8 – 14). All materials were administered daily to rats for 21 days. #p < 0.05 versus normal; *p < 0.05 versus negative control. Comparisons among different groups were analyzed using One-way ANOVA, followed by the Tukey’s post-hoc test.
4. Effect of SKE on the hippocampal AChE immunoreactive cell levels
AChE plays a biological role in the termination of impulse transmission at cholinergic synapses by rapidly hydrolyzing the neurotransmitter ACh to form acetate and choline (Čolović et al., 2013). AD is associated with a loss of cholinergic neurons in the brain, and the decreased levels of ACh, choline acetyltransferase and acetylcholinesterase (Lane et al., 2006).
To determine the AChE levels in the hippocampus, IHC staining was conducted in the normal, the control, and SKE-administered groups. AChE immune-reactive (AChE-ir) cell levels in the CA1 region of normal, control, SKE100, and SKE300 groups were 41.0 ± 3.0, 34.7 ± 1.9, 33.3 ± 3.9, and 55.7 ± 4.0, respectively. The AChE-ir cell levels in the CA3 region of normal, control, SKE100, and SKE300 groups were 51.2 ± 4.0, 27.5 ± 3.1, 45.6 ± 5.0, and 59.3 ± 7.4, respectively (p < 0.05). It was observed that the SKE300 group significantly attenuated the decrease in AChE-ir neurons in the CA3 region of the control group (p < 0.05, Fig. 4). The SKE100 group showed an increasing tendency in AChE-ir cells of the CA3 region.
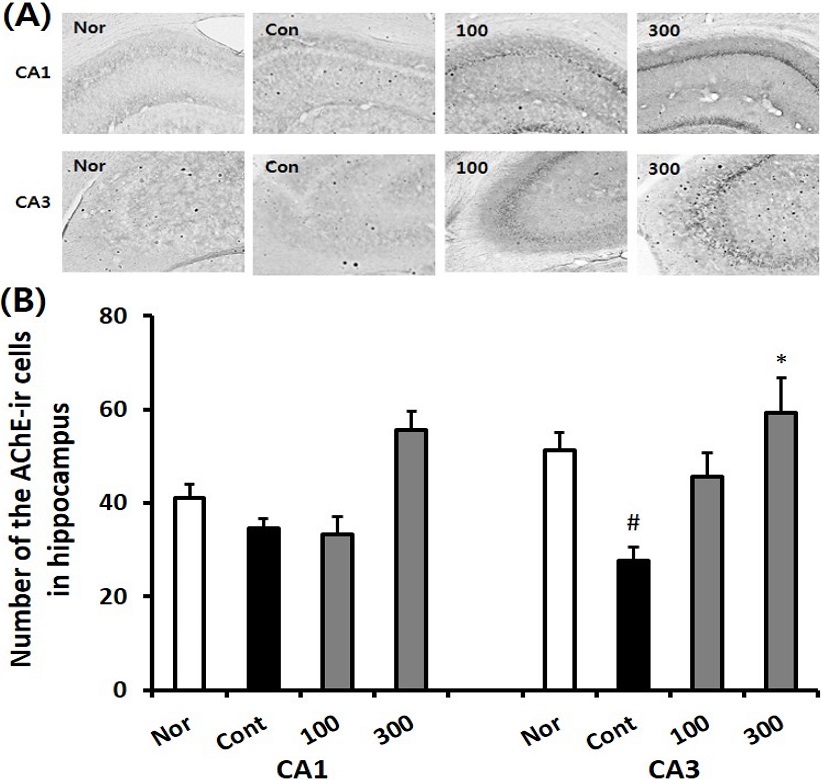
Effect of different concentrations of Sedum kamtschaticum leaf extract (SKE) administration on the number of AChE immuno-reactive cells in the hippocampal CA1 and CA3 regions after trimethyltin treatment by immunohistochemistry. The representative photographs and the number of the AChE immunoreactive cells are indicated in (A) and (B). Rats were randomly divided into four groups. Nor; normal, 0.9% saline days, Cont; negative control, 0.9% saline + trimethyltin 8 ㎎/㎏), SKE100; 100 ㎎/㎏ of Sedum kamtschaticum leaf extract in 0.9% saline + trimethyltin 8 ㎎/㎏), SKE300; 300 ㎎/㎏ of Sedum kamtschaticum leaf extract in 0.9% saline + trimethyltin 8 ㎎/㎏) (n = 8 – 14). All materials were administered daily to rats for 21 days. #p < 0.05 versus normal; *p < 0.05 versus negative control. Comparisons among different groups were analyzed using One-way ANOVA, followed by the Tukey’s post-hoc test.
In the article reported by Park et al. (2012), AChE-ir neurons of control in TMT-treated animal model was decreased than normal and those of the drug (squid phosphatidylserine) treated groups increased than control. In the present study, the hippocampal cells in the CA1 and CA3 regions of the control group were decreased compared to the normal group, but SKE treatment indicated a tendency for this to increase. SKE300 treatment significantly increased the AChE levels in the CA3 region. The increasing tendencies in AChE-immuno reactive cell level of SKE groups compared with those of control in CA3 region were also examined in the literature that Krill-derived phosphatidylserine group significantly alleviated the loss of acetylcholinergic neurons in the hippocampus and medial septum compared to that of the medium-chain triglyceride (MCT) vehicle group (Shim et al., 2012).
From AChE immunohistochemistry, SKE300 supplementation showed higher AChE activity in CA3 region. The result suggested that SKE300 could alleviate the dysfunction of cholinergic system affected by exposure to TMT.
5. Effect of SKE on the hippocampal BDNF immunoreactive cell level
BDNF is a growth factor in the nervous system, located in the cortex and hippocampus. It is transported from the entorhinal cortex to the hippocampus and is associated with memory ability (Lee et al., 2018). Decreased levels of BDNF in the hippocampus and partial cortex in the AD group compared with the control group, has been reported previously (Hock et al., 2000).
To investigate the BDNF levels in the hippocampus, IHC staining was conducted for the normal, the control and SKE-administered groups. The BDNF immune-reactive (BDNF-ir) cell level in the CA1 regions of normal, control, SKE100, and SKE300 groups were 189.5 ± 2.7, 186.4 ± 3.9, 189.8 ± 2.3, and 189.2 ± 2.6, respectively. The BDNF-ir cell level in the CA3 region of normal, control, SKE100, and SKE300 groups were 196.0 ± 6.0, 184.9 ± 3.6, 197.2 ± 2.8, and 193.2 ± 1.7, respectively. Based on the results, it can be concluded that the SKE100 and SKE300 groups showed an increasing tendency of BDNF-ir neurons in the CA3 region, compared with those of the control group (Fig. 5).
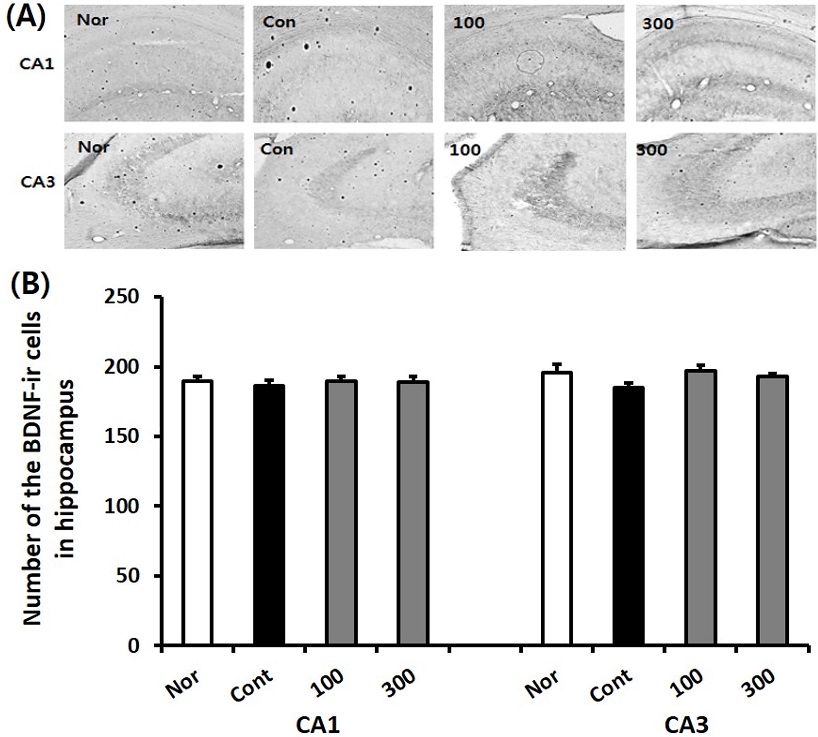
Effect of SKE administration on the BDNF immune-reactive cell levels in the hippocampus after TMT treatment by immunohistochemistry. The representative photographs and the number of the BDNF immunostained cells are indicated in (A) and (B). Rats were randomly divided into four groups (n = 8 - 14): Nor (normal, 0.9% saline days), Cont (negative control, 0.9% saline + TMT 8 ㎎/㎏), SKE100 (SKE 100 ㎎/㎏ in 0.9% saline + TMT 8 ㎎/㎏), and SKE300 (SKE 300 ㎎/㎏ in 0.9% saline + TMT 8 ㎎/㎏). All materials were administered aily to rats for 21 days. Comparisons among different groups were analyzed using One-way ANOVA, followed by the Tukey’s post-hoc test.
6. Effect of SKE on the hippocampal PKC immunoreactive cell levels
PKC plays a key role in learning and memory possibly by regulating synaptic plasticity in vertebrates. In mammalians, hippocampal PKC is activated by learning through various memory tasks (Noguès, 1997). TMT-induced neurotoxic damage is related to reactive oxygen species and reactive nitrogen species. TMT action is triggered by numerous molecular events and cellular pathways such as activation of various kinases including PKC, transcription factors, stress proteins, and early response genes (Geloso et al., 2011).
Measurement of the PKC immuno-reactive (PKC-ir) cells in the hippocampus was conducted in the normal, the control, and SKE-administered groups. The PKC-ir cell level in the CA1 region of the normal, control, SKE100, and SKE300 groups were 204.8 ± 1.5, 195.6 ± 1.6, 203.9 ± 3.3, and 202.4 ± 1.4, respectively. The PKC-ir cell level in the CA3 region of the normal, control, SKE100, and SKE300 groups were 196.8 ± 0.7, 186.5 ± 5.4, 202.6 ± 2.2, and 201.0 ± 2.0, respectively.
These results indicated that the SKE100 and SKE300 groups showed an increasing tendency of PKC-ir cells in the CA1 and CA3 regions, compared with the levels of the control group (Fig. 6).
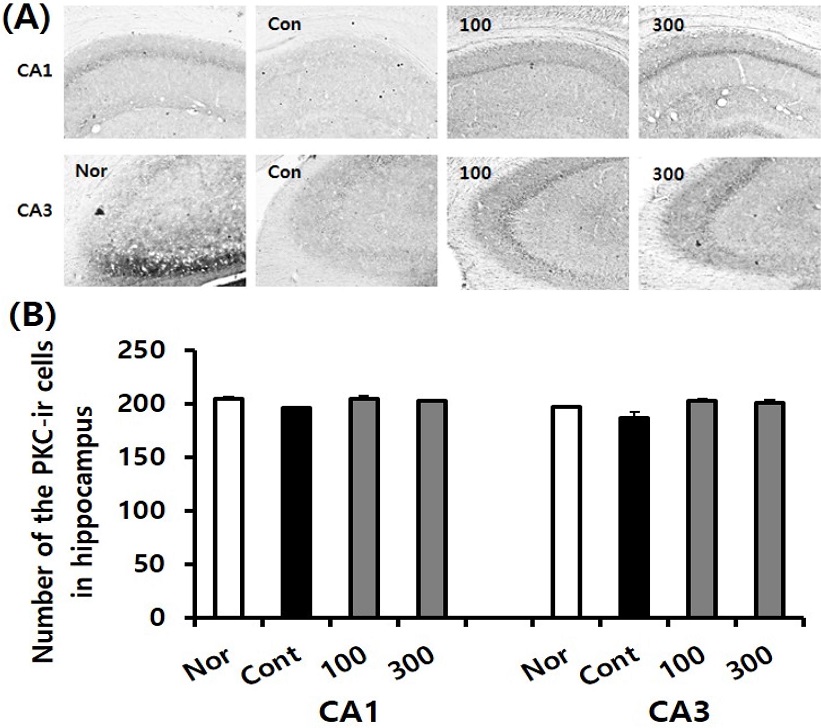
Effect of SKE administration on PKC immune-reactive cell levels in the hippocampus after TMT treatment by immunohistochemistry. The representative photographs and the number of the PKC immunostained cells are indicated in (A) and (B). Rats were randomly divided into four groups (n=8-14): Nor (normal, 0.9% saline days), Cont (negative control, 0.9% saline + TMT 8 ㎎/㎏), SKE100 (SKE 100 ㎎/㎏ in 0.9% saline + TMT 8 ㎎/㎏), and SKE300 (SKE 300 ㎎/㎏ in 0.9% saline + TMT 8 ㎎/㎏). All materials were administered aily to rats for 21 days. Comparisons among different groups were analyzed using One-way ANOVA, followed by the Tukey’s post-hoc test.
In the study, data showed that SKE showed more potent effect on AChE (20.0 - 54.3%) than BuChE (4.2 - 18.9%), which indicate that SKE could play a role in the regulation of synaptic acetylcholine level through AChE inhibition at AD.
The number of CREB-immunopositive neuronal cells in the hippocampus of TMT-treated rats with memory impairment, was significantly decreased compared with those in the control rats which were treated with saline instead of TMT (Lee et al., 2016).
In the present study, p-CREB immuno-reactive hippocampal cells of the control group were decreased compared to those of the normal group. However, the cells in SKE-treated groups showed a tendency to increase, and the SKE300 group showed significantly higher value than those of the control group.
And the result showed that SKE could play a role in enhancing the immune-reactive neuronal cell’s viability on AChE and could ameliorate memory deficit in TMT-treated rats. The hippocampal levels of BDNF and PKC in SKE groups indicated an increasing tendency compared to the levels in the control group, which were lower than those in the normal group.
In conclusion, these results suggest that with further research, SKE treatment could contribute to a novel treatment for cognitive improvement in AD.
Acknowledgments
This work was supported by a grant (PJ01193201) from the National Institute of Horticultural and Herbal Science, Rural Development Administration, Korea.
References
-
Chen H, Wu X, Gu X, Zhou Y, Ye L, Zhang K, Pan H, Wang J, Wei H, Zhu B, Naman CB, Mak S, Carlier PR, Cui W and Han Y. (2018). Tacrine(10)-hupyridone prevents post-operative cognitive dysfunction via the activation of BDNF pathway and the inhibition of AChE in aged mice. Frontiers in Cellular Neuroscience. 12:396. https://www.frontiersin.org/articles/10.3389/fncel.2018.00396/full, (cited by 2020 Oct 13).
[https://doi.org/10.3389/fncel.2018.00396]
-
Čolović MB, Krstić DZ, Lazarević-Pašti TD, Bondžić AM and Plátenı́k J, Kuramoto N and Yoneda Y. (2000). Molecular mechanisms associated with long-term consolidation of the NMDA signals. Life Sciences. 67:335-364.
[https://doi.org/10.1016/S0024-3205(00)00632-9]
-
Rinne JO, Kaasinen V, Järvenpää T, Någren K, Roivainen A, Yu M, Oikonen V and Kurki T. (2003). Brain acetylcholinesterase activity in mild cognitive impairment and early alzheimer’s disease. Journal of Neurology, Neurosurgery, and Psychiatry. 74:113-115.
[https://doi.org/10.1136/jnnp.74.1.113]
-
Sadeghi L, Yousefi Babadi V and Tanwir F. (2018). Improving effects of Echium amoenum aqueous extract on rat model of alzheimer's disease. Journal of Integrative Neuroscience. 17:661-669.
[https://doi.org/10.3233/JIN-180093]
-
Saleem U, Raza Z, Anwar F, Ahmad B, Hira S and Ali T. (2019). Experimental and computational studies to characterize and evaluate the therapeutic effect of Albizia lebbeck(L.) seeds in alzheimer’s disease. Medicina. 55:184. https://www.mdpi.com/1010-660X/55/5/184, (cited by 2020 Sep 13).
[https://doi.org/10.3390/medicina55050184]
-
Shim HS, Park HJ, Ahn YH, Her S, Han JJ, Hahm DH, Lee HJ and Shim IS. (2012). Krill-derived phosphatidylserine improves TMT-induced memory impairment in the rat. Biomolecules and Therapeutics. 20:207-213.
[https://doi.org/10.4062/biomolther.2012.20.2.207]
-
Singhal G, Jaehne EJ, Corrigan F, Toben C and Baune BT. (2014). Inflammasomes in neuroinflammation and changes in brain function: A focused review. Frontiers in Neuroscience. 8:315. https://www.frontiersin.org/articles/10.3389/fnins.2014.00315/full, (cited by 2020 Sep 13).
[https://doi.org/10.3389/fnins.2014.00315]
-
Tabet N. (2006). Acetylcholinesterase inhibitors for alzheimer’s disease: Anti-inflammatories in acetylcholine clothing. Age and Ageing. 35:336-338.
[https://doi.org/10.1093/ageing/afl027]
-
Talesa VN. (2001). Acetylcholinesterase in alzheimer’s disease. Mechanisms of Ageing and Development. 122:1961-1969.
[https://doi.org/10.1016/S0047-6374(01)00309-8]
-
West MJ, Coleman PD, Flood DG and Troncoso JC. (1994). Differences in the pattern of hippocampal neuronal loss in normal ageing and alzheimer's disease. The Lancet. 344:769-772.
[https://doi.org/10.1016/S0140-6736(94)92338-8]
-
Ye MS, Han BH, Kim JS, Kim KS and Shim IS. (2020). Neuroprotective effect of bean phosphatidylserine on TMT-induced memory deficits in a rat model. International Journal of Molecular Sciences. 21:4901. https://www.mdpi.com/1422-0067/21/14/4901, (cited by 2020 Sep 30).
[https://doi.org/10.3390/ijms21144901]
-
Ye MS, Lee SG, Chung ES, Lim SJ, Kim WS, Yoon HR, Kim SK, Ahn KS, Jang YP and Bae HS. (2014). Neuroprotective effects of Cuscutae semen in a mouse model of Parkinson’s disease. Evidence-Based Complementary and Alternative Medicine. 2014:150153. https://www.hindawi.com/journals/ecam/2014/150153/, (cited by 2020 Sep 13).
[https://doi.org/10.1155/2014/150153]
-
Zhang C, Xu Q, Xiao X, W, Kang Q, Zhang X, Wang T and Li Y. (2018). Prenatal deltamethrin exposure-induced cognitive impairment in offspring is ameliorated by memantine through NMDAR/BDNF signaling in hippocampus. Frontiers in Neuroscience. 12:615. https://www.frontiersin.org/articles/10.3389/fnins.2018.00615/full, (cited by 2020 Sep 13).
[https://doi.org/10.3389/fnins.2018.00720]