
엽록체 유전체 바코딩기술 및 HPLC를 이용한 복분자딸기 식별법
This is an open access article distributed under the terms of the Creative Commons Attribution Non-Commercial License (http://creativecommons.org/licenses/by-nc/3.0/) which permits unrestricted non-commercial use, distribution, and reproduction in any medium, provided the original work is properly cited.
Abstract
Rubus coreanus and R. occidentalis are commonly sold as, ‘Bokbunja’, in the Korean market. However, according to Korean Pharmacopoeia, ‘Bokbunja’ refers to the unripened fruits of R. coreanus. Therefore, authentication of R. coreanus and its related species is necessary to prevent adulteration and improper use.
Genomic DNA was extracted from leaves, stem and fruits of R. coreanus, fruits of R. occidentalis and leaves of R. longisepalus and R. hirsutus. The anthocyanin profile of R. coreanus and R. occidentalis was identified by high performance liquid chromatography/diode-array detection. We designed four universal barcoding primer sets with optimal restriction enzyme sites to identify polymerase chain reaction-restriction fragment length polymorphisms (PCRRFLPs) between R. coreanus, the newly assembled chloroplast genome of R. occidentalis, and two related species. In addition, two Insertion or Deletion (InDel) markers were also developed. These markers were successfully used to distinguish each species in nine samples.
In this study, we sequenced and assembled the complete chloroplast genome of R. occidentalis and developed a DNA barcoding method to differentiate it from other related species. Thus, anthocyanin profiling, PCR-RFLPs, and InDel markers are useful authentication tools to distinguish R. coreanus from R. occidentalis.
Keywords:
Rubus coreanus, Chloroplast, Insertion or Deletion Marker, PCR-RFLP, Single Nucleotide Polymorphism서 언
산딸기 속 (Rubus)은 장미과에 속하며 약 300 여 종에 이르는 것으로 알려져 있다. 우리나라에는 300 여 종 중 22 종이 보고되어 있으며 그 중 10 종 이상이 식용으로 사용되고 있다 (NIFDS, 2018).
복분자딸기 (Rubus coreanus Miq.)는 우리나라에서 대표적으로 많이 이용되는 산딸기 속 식물의 하나로 예로부터 그 효능으로 인해 약용뿐만 아니라 복분자주와 같이 식용으로도 많이 이용되고 있다. 대한민국약전에 따르면 복분자딸기의 생약소재는 복분자딸기의 덜 익은 열매를 의미한다 (MFDS, 2019). 하지만 중국과 대만 약전에서는 화동복분자 (Rubus chingii Hu.)를 복분자의 기원 식물로 하고 있으며 북한 약전에서는 복분자딸기와 함께 산딸기 (Rubus crataegifolius Bunge.)도 복분자의 기원 식물로 규정하고 있다 (NIFDS, 2018).
동아시아 지역에서 복분자의 기원 식물로 규정되는 여러 근연식물뿐만 아니라 캐나다 원산의 블랙라즈베리 (Rubus occidentalis L.) 역시 시장에서 복분자라는 동일한 이름으로 유통되고 있다. DNA 마커에 기반한 조사 결과, 시장이나 온라인상에서 복분자로 판매되는 상당수가 복분자딸기의 근연종으로 판별된 바 있다 (NIFDS, 2018; Mohanan et al., 2019). 따라서 유통상의 혼란을 방지하고 식품과 의약품의 위변조 및 품질관리를 위해 복분자를 명확히 감별하는 것은 매우 중요하다.
DNA 수준에서 식물의 종 판별을 위해 많이 사용되는 식물의 유전체 지역은 엽록체이다. 엽록체는 많은 식물에서 모계유전을 하는 것으로 알려져 있고, 모계유전이라는 특성으로 인해 유전체에 재조합이 거의 일어나지 않는다 (Greiner et al., 2015). 따라서 많은 식물에서 엽록체 유전체의 크기, 유전자의 수와 구성 등이 잘 보존되어 있다. 하지만 이러한 특성에도 종간 식별이 가능할 수준의 변이가 축적되는 진화속도를 가지고 있어 종 판별이나 계통분석에 많이 이용된다 (Palmer, 1985; Wolfe et al., 1987; Wicke et al., 2011; Li et al., 2015).
이러한 엽록체 유전체의 변이지역에 기반하여 한약재와 같이 오용 가능성이 있는 식물들에 대한 감별법 등이 복분자를 비롯해 여러 식물에서 개발된 바 있다 ( Park et al., 2017; Kim et al., 2018; NIFDS, 2018; Mohanan et al., 2019; Jeong et al., 2021). 엽록체에 존재하는 다양한 종류의 유전체 서열정보가 동일 식물의 미토콘드리아 유전체에서 매우 잘 보존된 형태로 존재할 수 있으며 이러한 DNA 영역을 mitochondrial plastid DNA (MTPT)라고 한다. 이는 식물에 따라 매우 다양한 형태로 존재하며 엽록체 기반의 식물 동정 과정에 오동정을 유발할 수 있다 (Sloan and Wu, 2014; Park et al., 2020). 또한 최근 동일 종내 많은 자원에서 엽록체 유전체를 완전히 완성하고 비교하게 되면서 종내 엽록체 변이도 상당히 존재하는 것이 발견되었다 (Kim et al., 2015; Kim et al., 2017; Kang et al., 2018). 따라서 엽록체 기반 유전자 분석법 개발과정에서 MTPT에 의한 오동정 가능성을 고려함과 동시에 종내 변이에 대한 사항도 고려하는 것이 필요하다.
복분자딸기 및 블랙라즈베리 과실의 안토시안류 HPLC 프로파일링과 관련한 기존 연구에 따르면, 복분자딸기에서는 cyanidin-3-glucoside (C3G)을 높게 함유하고 있음이 보고된 바 있으나, 블랙라즈베리에서는 cyanidin-3-rutinoside (C3R)이 주요한 안토시안으로 보고된 바 있다 (Tulio et al., 2008; Lee et al., 2013; Lee et al., 2014).
본 연구에서는 복분자딸기 및 블랙라즈베리 과실의 cyanidin류 중 cyanidin-3-sambubioside (C3S), C3G 및 C3R 표준물질을 이용하여, 정성 및 정량 분석을 실시하였으며, 궁극적으로는 건강기능식품 소재로 원료 활용 시 품종 확인 및 혼입방지를 위한 분석법으로서의 활용 가능성을 확인하고자 하였다.
더불어 복분자와 블랙라즈베리와 근연종을 구별하는 유전자분석법 개발을 동시에 수행하였다. 이를 위해 NCBI와 같은 공공 데이터베이스에서 획득한 산딸기 속 식물들의 엽록체 서열과 본 연구에서 완성한 블랙라즈베리의 엽록체 완전장을 바탕으로 변이를 발굴하였다. 그 중 4 개의 universal 바코딩 지역 primer 세트에서 증폭된 PCR 증폭 산물에 제한효소를 처리하여 PCR 증폭 산물의 restriction fragment length polymorphism (RFLP)를 확인하는 PCR-RFLP 방법과 증폭된 PCR 증폭 산물의 크기 차이를 이용하는 insertion or deletion (InDel) 마커 2 개를 사용해 엽록체 유전체 기반의 복분자딸기 식별법을 검증하였다.
본 연구에서 개발된 4개의 universal primer 및 제한효소세트, 2 개의 InDel 마커, cyanidin류 프로파일 분석을 통해 확인된 복분자딸기와 블랙라즈베리의 cyanidin류 차이는 복분자딸기와 블랙라즈베리 및 근연식물의 감별법 확립 및 복분자 딸기와 블랙라즈베리를 이용한 식품 산업의 발전에 큰 도움을 줄 것이다.
재료 및 방법
1. 시료 수집
본 연구에서는 다음과 같은 자원들을 수집하였다 (Table 1). 복분자딸기 (Rubus coreanus Miq.) 5 개체와 블랙라즈베리 (Rubus occidentalis L.) 1 개체를 포함하여 거제딸기, 맥도딸기, 장딸기 1 개체도 비교분석을 위해 수집되었다. 복분자딸기의 줄기는 횡성 농가에서, 잎은 국립생물자원관에서 제공 받았으며, 블랙라즈베리의 열매는 베리앤바이오식품연구소에서, 거제딸기, 맥도딸기, 장딸기의 잎은 한택식물원에서 분양받아 사용하였다.
2. DNA 추출
수집된 개체들의 조직은 액체질소를 이용하여 급속냉각을 시킨 후 막자사발을 이용하여 분쇄하였다. 분쇄하기 전 측정한 조직의 양이 0.4 g보다 많은 경우 Exgene Plant SV Midi Kit (Geneall Biotechnology Co., Ltd., Seoul, Korea)를 사용하였고, 0.4 g보다 적으면 Exgene Plant SV Mini Kit (Geneall Biotechnology Co., Ltd., Seoul, Korea)를 이용하여 제조사에서 제공하는 프로토콜에 따라 DNA를 추출하였다.
추출된 DNA의 농도는 0.8% agarose gel과 Nanodrop 2000 (Thermo Fisher Scientific Inc., Wilmington, DE, USA)을 통해 확인한 후 distilled water를 이용해 10 ng/㎕로 희석하였다.
3. 엽록체 완전장 조립
추출된 블랙라즈베리의 DNA는 Illumina Miseq platform을 통해 paired-end library로 시퀀싱되어 약 1.07 Gb의 데이터를 생산하였다. 생산된 서열 정보와 본 연구실에서 개발한 엽록체 유전체 조립 기술인 de novo assembly of low-coverage whole-genome sequencing (dnaLCW) 방법을 이용해 블랙라즈베리의 엽록체 완전장을 조립하였다 (Kim et al., 2015). 조립된 엽록체 완전장 서열의 유전자 부위들은 GeSeq (https://chlorobox.mpimp-golm.mpg.de/geseq.html)과 a rtemis를 통해 부여하였다 (Carver et al., 2012; Tillich et al., 2017). 산딸기 4 종의 엽록체 지도는 OrganellarGenomeDRAW (OGDRAW)를 통해 작성하였다 (Greiner et al., 2019).
4. 엽록체 완전장 서열 비교
수집한 4 개 종을 식별하고자 새로 완성한 블랙라즈베리 외에 본 연구실에서 완성한 거제딸기 (R. longisepalus, MW436703)와 장딸기 (R. hirsutus, MW448480)를 비롯해 복분자딸기 (R. coreanus, NC_042715.1)의 엽록체 완전장 서열을 수집하였다.
MAFFT Web Version (https://mafft.cbrc.jp/alignment/server/index.html)을 통해 수집한 엽록체 서열들을 alignment하였다. Alignment된 결과를 바탕으로 확인된 변이지역들은 Primer-BLAST (https://www.ncbi.nlm.nih.gov/tools/primer-blast/)를 통해서 마커로 개발될 수 있는지 확인하였다.
5. 바코딩 지역에 기반한 RFLP와 InDel 마커 적용
식물의 종 판별에서 바코딩 지역으로 많이 사용되는 엽록체의 matK, rbcL, trnH-psbA, trnL-trnF 총 4 지역을 universal primer를 통한 PCR-RFLP를 위한 증폭 지역으로 선택하였으며 alignment하였을 때 식별 가능한 길이 차이를 보인 2 지역을 InDel 마커를 위한 증폭 지역으로 선택하였다.
수집한 4 종의 산딸기 속 식물의 엽록체 서열과 Primer-BLAST를 이용해 universal primer와 InDel 마커로 생성될 PCR 증폭물의 서열을 예측하였다. In silico 상에서 예측된 universal primer의 PCR 증폭물 서열은 NEBcutter V2.0 (Vincze et al., 2003; https://nc2.neb.com/NEBcutter2/)를 통해 절단물의 크기 차이를 발생시키는 특정 제한 효소들을 예측하였다. 이후 실제 실험을 통해 annealing 온도를 비롯하여 universal primer에서 제시하는 PCR 조건을 사용하여 4 가지 universal primer 세트의 PCR 증폭물을 생성하였다. InDel 마커는 pre-denaturation 94℃ 10 분, denaturation 94℃ 20 초, annealing 54℃ 20 초, extension 72℃ 20 초 35 회 반복하였으며 final extension 72℃ 10 분의 조건으로 PCR 증폭물을 생성하였다. 4 개의 universal primer PCR 증폭물에 제한 효소를 처리하기 전에 2.0% agarose gel을 통해 in silico 결과와 일치하는지 확인하였다.
Agarose gel을 통해 확인한 PCR 증폭물의 결과와 in silico 결과가 일치하는 것을 확인한 후 PCR 증폭물 5.0 ㎕, distilled water 8.3 ㎕, 제한효소 0.2 ㎕ (ClaI; AciI; MboI; RsaI, New England Biolabs, Ipswich, MA, USA), 제한효소 buffer 1.5 ㎕ (CutSmart® Buffer, New England Biolabs, Ipswich, MA, USA)로 제한효소를 포함한 mixture를 만들었고, 이를 37℃에서 4 시간 동안 incubation 시켰다. 이후 제한효소 mixture의 절단 패턴과 InDel 마커 PCR 증폭물의 크기 차이를 2.5% agarose gel에서 전기영동하여 확인하였다.
6. Cyanidin 배당체 분석
Cyanidin 배당체 분석은 기존 보고된 분석 방법을 일부 수정하여 사용하였다 (Tulio et al., 2008; Lee et al., 2013).
시험용액은 100 g의 복분자딸기 과실 (Hoengseong, Korea) 및 블랙라즈베리 과실 (Gochang, Korea)을 1 ℓ의 50% 주정으로 히팅 맨틀을 이용하여 80℃에서 2 시간 동안 2 회 가열추출하여, 농축한 후 동결 건조하여 사용하였다. 균질화된 1 g을 취하여 희석용매 (0.1%, trifluoroacetic acid in methanol)를 10 ㎖을 가하여 10 분간 sonication 한 후, 0.45 ㎛ 실린지 필터로 여과하여 사용하였다.
분석은 HPLC (Agilent 1260, Agilent Technologies Inc., Santa Clara, CA, USA)를 사용하여 d iode a rra y detector (DAD) 파장 520 ㎚에서 정량분석하였다. 이동상은 5% formic acid와 100% 메탄올을 사용하여 g ra dient 조건을 적용하였다. 정량을 위한 표준품은 cyanidin-3-O-sambubioside chloride (CFN92173 98%, ChemFaces, Wuhan, China), cyanidin-3-O-glucoside chloride (CFN99740 98%, ChemFaces, Wuhan, China), cyanidin-3-O-rutinoside chloride (CFN92135 98%, ChemFaces, Wuhan, China)를 사용하였다.
결과 및 고찰
1. 블랙라즈베리의 엽록체 완전장 서열
본 연구에서는 엽록체 조립 기술인 dnaLCW를 이용하여 블랙라즈베리 (Rubus occidentalis L.)의 엽록체 완전장을 완성하였다. 완성된 블랙라즈베리의 엽록체는 156,253 bp의 크기를 가졌으며, 엽록체를 구성하는 4 개 부위인 Long Single Copy (LSC)는 85,889 bp, Short Single Copy (SSC)는 18,862 bp, 두개의 Inverted Repeat (IR)는 25,751 bp로 구성되었다.
엽록체를 구성하고 있는 유전자는 85 개의 protein coding gene, 37 개의 tRNA, 8 개의 rRNA를 가지고 있으며 이는 본 연구실에서 이전에 완성한 근연종 식물인 거제딸기 및 장딸기와 같은 구조 및 유전자 수이다 (Park et al., 2021).
2. 엽록체 서열 비교 결과
수집한 엽록체 4 종을 비교한 결과 엽록체 전체의 크기는 155,405 bp - 156,253 bp로 나타났으며 동일한 유전자 구성을 확인하였다 (Fig. 1 and Table 2).
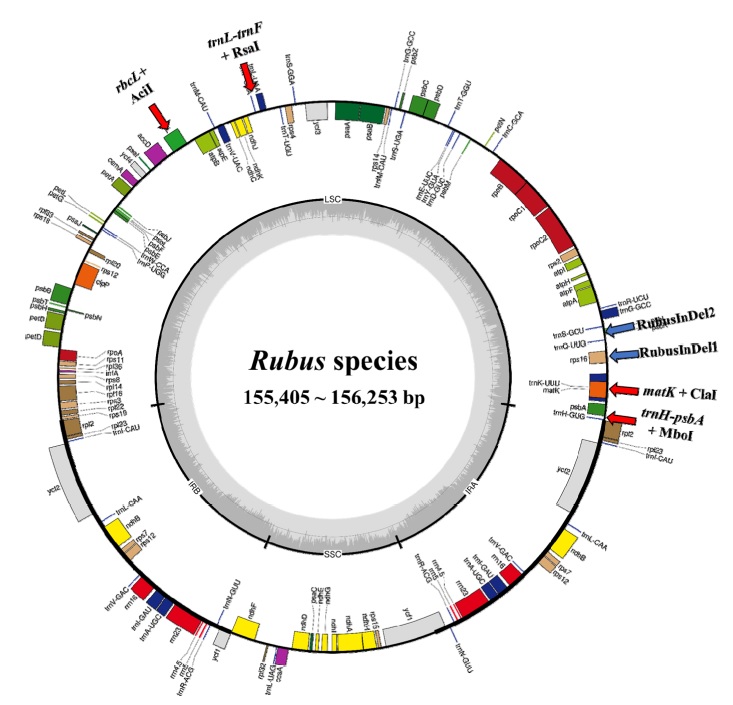
Chloroplast genome map of four Rubus species.Red arrow indicates universal primer amplifying region. Blue arrow indicates InDel marker amplifying region.
엽록체를 구성하는 4 개 부위인 LSC, SSC, 2 개의 IR을 살펴보면, LSC는 85,322 bp - 85,889 bp, SSC는 17,693 bp - 18,862 bp, IR은 25,751 bp - 26,195 bp의 크기를 가지는 것으로 나타났다. 블랙라즈베리가 156,253 bp로 가장 긴 서열을 가진 반면 복분자딸기 (Rubus coreanus Miq.)는 155,405 bp로 가장 짧은 서열을 가지는 것으로 나타났다. MAFFT로 alignment된 서열들을 비교했을 때, 총 3,886 개의 SNP와 861 개의 InDel 지역들이 확인되었다 (Table 3).
개별 종간 일대일 비교하였을 때는 1,157 개 - 2,558 개의 SNP와 273 개 - 469 개의 InDel 지역들이 존재하는 것으로 확인되었다. 복분자딸기와 블랙라즈베리 간에 변이지역이 가장 적은 것으로 나타났고, 복분자딸기와 장딸기 간에 변이지역이 가장 많은 것으로 나타났다. 이는 선행연구에서 확인된 거제딸기, 장딸기, 복분자딸기, 블랙라즈베리 간 유연관계의 거리가 반영된 결과로 보인다 (Oka da et al., 2020; Park et al., 2021).
3. PCR-RFLP를 이용한 복분자 근연종 식별법
엽록체 유전체 중 4 개의 바코딩 타겟을 증폭하는 universal primer를 이용해 PCR 증폭한 후 4 개 종 모두 식별되는 제한효소 조합을 선정하였다. 각각의 PCR 증폭 산물을 대상으로 최적의 제한효소 조합을 선정하고 in silico 상에서 각각의 절단 산물을 예측하였을 때 4 개 종의 식별이 가능하였으며 2 개의 InDel 마커를 통해서도 복분자딸기를 식별할 수 있었다 (Fig. 2 and Table 4).
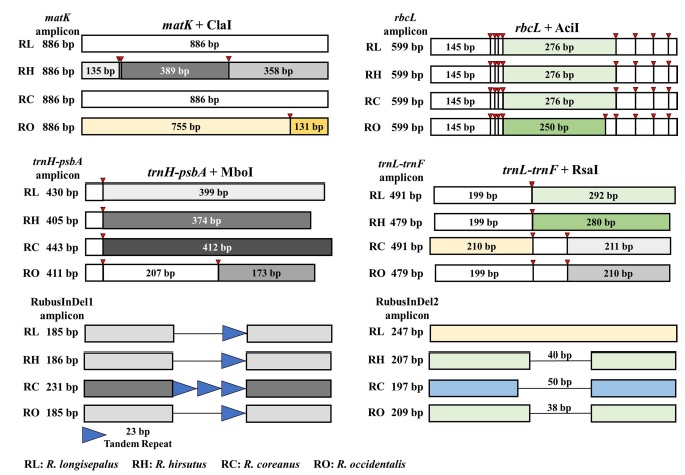
RFLP and InDel fingerprinting pattern of Rubus species.Value makred left of the bar is PCR amplicon size. Red arrow indicates enzyme cutting site to the PCR amplicon. Product size under 100 bp were not marked.
이후 실제 9 개체의 DNA를 증폭시킨 결과, 4 개의 universal primer와 2 개의 InDel 마커를 통해 matK에서 맥도딸기 개체를 제외한 8 개체가 증폭되었고, rbcL, trnH-psbA, trnL-trnF, 2 개의 InDel 마커에서는 9 개체 모두 증폭물이 형성된 것을 확인하였다. 이후 제한효소를 처리하여 PCR 증폭물과 제한효소 mixture의 절단 패턴 및 InDel 마커에 의한 크기 차이를 agarose gel을 통해 확인하였다 (Fig. 3).
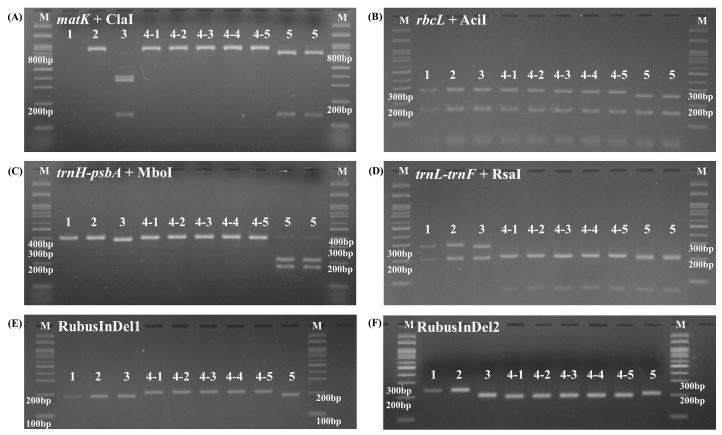
Screening of Rubus collections using PCR-RFLPs and InDel markers.M is 100 bp ladder. (A) matK with ClaI. R. longisepalus and R. coreanus were not digested by restriction enzyme. R. hirstus and R. occidentalis showed different digested pattern. (B) rbcL with AciI. Restriction pattern of R. longisepalus, R. hirsutus and R. coreanus is different with R. occidentalis. (C) trnH-psbA with MboI. R. longisepalus, R. hirsutus and R. coreanus showed one band while R. occidentalis showed two band. (D) All the four species showed different band pattern. (E) RubusInDel1 marker result. R. coreanus showed larger band size than other species. (F) RubusInDel2 marker result. R. coreanus showed smaller band size than other species.
바코딩 마커 4 개의 지역을 제한효소로 절단하였을 때, matK의 경우 거제딸기와 복분자딸기에서는 절단이 나타나지 않아 886 bp의 증폭물을 나타냈고, 장딸기는 135 bp, 358 bp, 389 bp의 3 가지 밴드를 나타냈으며, 블랙라즈베리는 131, 755 bp로 2 가지 밴드 패턴을 보였다. 또한, rbcL의 경우 맥도딸기, 거제딸기, 장딸기, 복분자딸기는 145 bp, 276 bp의 2 가지 밴드를 나타냈으며, 블랙라즈베리는 145 bp, 250 bp의 2 가지 밴드를 보였다. trnH-psbA에서 맥도딸기와 거제딸기는 399 bp, 장딸기는 374 bp, 복분자딸기는 412 bp의 1 가지 밴드가 나타났으며, 블랙라즈베리는 173 bp, 207 bp의 2 가지 밴드를 보였다. 그리고 trnL-trnF의 경우 맥도딸기와 거제딸기는 199 bp, 292 bp, 장딸기는 199 bp, 280 bp로 각각 2 가지 밴드가 나타났지만 복분자딸기는 211 bp의 1 가지 밴드를 보였다. 블랙라즈베리는 199 bp, 210 bp의 2 가지 밴드가 예측되었으나 증폭물의 크기 차이로 인해 agarose gel상에서 식별이 어려운 것으로 나타냈다. RubusInDel1 마커에서 맥도딸기, 거제딸기, 장딸기, 블랙라즈베리에서는 185 bp, 186 bp의 해당하는 밴드가 나타났고, 복분자딸기에서는 231 bp의 밴드가 나타났다. RubusInDel2 마커에서는 맥도딸기와 거제딸기는 247 bp, 장딸기는 207 bp, 복분자딸기는 197 bp, 블랙라즈베리는 209 bp의 밴드를 나타냈다. 제한효소로 절단된 PCR 증폭물들과 InDel 마커로 생성된 증폭물들의 크기가 in silico 결과와 일치하는 것을 확인하였으며 복분자딸기와 블랙라즈베리의 식별뿐 아니라 실험에 사용된 4 개의 식물 종 모두 식별 가능한 것을 확인하였다.
이와 더불어 본 감별법에서 사용되지는 않았으나 복분자딸기의 또 다른 근연종인 화동복분자와 산딸기의 NCBI 등록 서열을 획득하여 앞서 수행한 4 개의 universal primer 및 제한효소 세트 및 InDel 마커 사용 했을 때 예측한 결과, trnH-psbA로 화동복분자와 산딸기 개체 간 식별이 가능할 것으로 예측되었고, matK, rbcL, trnL-trnF를 이용해 복분자딸기, 블랙라즈베리와 식별이 가능할 것으로 예측되었다. 또한 2 개의 InDel 마커에서 복분자딸기가 특이적으로 식별될 것으로 예측되었다.
4. Cyanidin 배당체 HPLC 분석 결과
Cyanidin 배당체류를 HPLC를 이용하여 분석한 결과, 복분자딸기의 주요한 안토시안은 C3G (전체 검출 피크 중 약 82.2 area %) 및 C3R (전체 검출 피크 중 약 1 7.8 a rea %) 순으로 구성되어 있음을 확인하였다 (Fig. 4A).
이는 기존연구에서 확인한 복분자딸기의 주요한 안토시안인 C3G 및 C3R 순서와 동일하였다 (Lee et al., 2013; Lee et al., 2014). 반면, 블랙라즈베리의 주요한 안토시안은 C3R (전체 검출 피크 중 약 56.0 area %)로 확인 되어 기존연구와 동일하게 나타났다 (Fig. 4B) (Tulio et al., 2008).
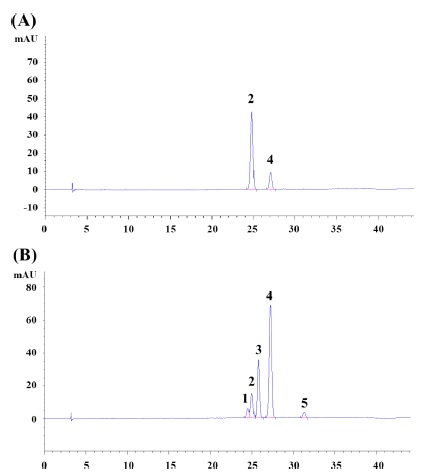
HPLC Chromatogram of R. coreanus and R. occidentalis fruits.Peak name; 1. Cyanidin-3-sambubioside, 2. Cyanidin- 3-glucoside, 3. unknown peak, 4. Cyanidin-3-rutinoside, 5. unknown peak; (A) R. coreanus Fruit, (B) R. occidentalis Fruit.
또한, C3S, C3G 및 C3R 표준품을 이용하여, 정량분석을 실시하였다. 그 결과, 복분자딸기는 C3G 3.7% 및 C3R 0.53%의 안토시안을 함유하고 있었으며, 블랙라즈베리는 C3R 3.80%, C3G 1.26%, C3S 0.95%의 안토시안을 함유하고 있어 복분자딸기와 블랙라즈베리의 cyanidin 배당체 구성과 함량이 명확하게 차이가 있음을 확인할 수 있었다.
본 연구에서 개발한 4 개의 PCR-RFLP 세트와 2 개의 InDel 마커를 이용해 복분자딸기와 근연종간 식별이 가능하였다. 또한 복분자딸기의 다른 근연종과의 식별 역시 가능할 것으로 예측된다. 뿐만 아니라 엽록체 유전체 연구를 통해 산딸기 속 식물 4 종의 서열 간에 많은 변이가 누적되어있음을 확인할 수 있었다. 따라서 추가 연구를 통해 다양한 분자 마커들을 개발하여 복분자딸기와 국내에서 식용으로 이용되는 10 여종의 산딸기류 식물들의 동정도 가능하리라 판단할 수 있다.
본 연구에서는 분자마커를 이용한 식별 뿐 아니라 HPLC를 이용한 분석법을 통해서도 복분자딸기 및 블랙라즈베리 진위판별이 가능함을 확인하였다. 따라서 분자마커를 이용한 식별법과 HPLC를 통한 성분 분석 식별법이 상호보완적으로 작용하여 건강기능식품 원재료 활용 시 품종 확인 및 혼입방지를 위한 분석법으로 활용 가능할 것으로 사료된다.
Acknowledgments
본 연구는 한국식품산업클러스터진흥원 2020 국산소재 기능성 규명사업의 지원을 받아 수행되어졌으며 이에 감사드립니다.
References
-
Carver T, Harris SR, Berriman M, Parkhill J and McQuillan JA. (2012). Artemis: An integrated platform for visualization and analysis of high-throughput sequence-based experimental data. Bioinformatics. 28:464-469.
[https://doi.org/10.1093/bioinformatics/btr703]
-
Greiner S, Lehwark P and Bock R. (2019). OrganellarGenome DRAW(OGDRAW) version 1.3.1: Expanded toolkit for the graphical visualization of organellar genomes. Nucleic Acids Research. 47:59-64.
[https://doi.org/10.1093/nar/gkz238]
-
Greiner S, Sobanski J and Bock R. (2015). Why are most organelle genomes transmitted maternally? Bioassays. 37:80-94.
[https://doi.org/10.1002/bies.201400110]
-
Jeong HJ, Lee JB, Gil JS, Hong CP, Kang SH, Kwon SJ, Kim HJ, Kim CK, Lee JH and Lee Y. (2021). Development of chloroplast-based InDel markers for the discrimination of Schisandraceae plant species. Korean Journal of Medicinal Crop Science. 29:11-16.
[https://doi.org/10.7783/KJMCS.2021.29.1.11]
-
Kang SJ, Park HS, Koo HJ, Park JY, Lee DY, Kang KB, Han SI, Sung SH and Yang TJ. (2018). The complete chloroplast genome sequence of Korean Lonicera japonica and intra-species diversity. Mitochondrial DNA Part B. 3:941-942.
[https://doi.org/10.1080/23802359.2018.1502637]
-
Kim IS, Park JY, Lee YS, Joh HJ, Kang SJ, Murukarthick J, Lee HO, Hur YJ, Kim Y, Kim KH, Lee SC and Yang TJ. (2017). The complete chloroplast genome sequence and intra-species diversity of Rhus chinensis. Plant Breeding and Biotechnology. 5:243-251.
[https://doi.org/10.9787/PBB.2017.5.3.243]
-
Kim KH, Lee SC, Lee JK, Lee HO, Joh HJ, Kim NH, Park HS and Yang TJ. (2015). Comprehensive survey of genetic diversity in chloroplast genomes and 45S nrDNAs within Panax ginseng species. PLoS ONE. 10:e0117159. https://journals.plos.org/plosone/article?id=10.1371/journal.pone.0117159, (cited by 2021 Sep 24).
[https://doi.org/10.1371/journal.pone.0117159]
-
Kim YS, Park HJ, Lee DH and Kim HK. (2018). Development of multiplex polymerase chain reaction assay for identification of Angelica species. Korean Journal of Medicinal Crop Science. 26:26-31.
[https://doi.org/10.7783/KJMCS.2018.26.1.26]
-
Kress WJ, Erickson DL, Jones FA, Swenson NG, Perez R, Sanjur O and Bermingham E. (2009). Plant DNA barcodes and a community phylogeny of a tropical forest dynamics plot in Panama. Proceedings of the National Academy of Sciences. 106:18621-18626.
[https://doi.org/10.1073/pnas.0909820106]
-
Lee JM, Dossett M and Finn CE. (2013). Anthocyanin fingerprinting of true bokbunja(Rubus coreanus Miq.) fruit. Journal of Functional Foods. 5:1985-1990.
[https://doi.org/10.1016/j.jff.2013.06.006]
-
Lee JM, Dossett M and Finn CE. (2014). Mistaken identity: Clarification of Rubus coreanus Miquel(Bokbunja). Molecules. 19:10524-10533. https://www.mdpi.com/1420-3049/19/7/10524, (cited by 2021 Sep 24).
[https://doi.org/10.3390/molecules190710524]
-
Li X, Yang Y, Henry RJ, Rossetto M, Wang Y and Chen S. (2015). Plant DNA barcoding: From gene to genome. Biological Reviews. 90:157-166.
[https://doi.org/10.1111/brv.12104]
- Ministry of Food and Drug Safety(MFDS). (2019). Korean Pharmacopoeia. Ministry of Food and Drug Safety. Cheongju, Korea. p.49-50.
-
Mohanan P, Hurh J, Kim SO, Na JR, Kim YJ, Lee HA, Kim MK and Yang DC. (2019). Chloroplast DNA-derived markers for the authentication of oriental medicinal Rubus species and mistaken identity of bokbunja in the local markets of Korea. Plant Biotechnology Reports. 13:305-314.
[https://doi.org/10.1007/s11816-019-00540-5]
- National Institute of Food and Drug Safety Evaluation(NIFDS). (2018). Disrcirmination of Rubus fruit. National Institute of Food and Drug Safety Evaluation. Cheongu, Korea. p.5
-
Okada A, Kikuchi S, Hoshino Y, Kunitake H and Mimura M. (2020). Phylogeny and trait variation of Japanese Rubus subgenus Ideaobatus. Scientia Horticulturae. 264:109150. https://www.sciencedirect.com/science/article/pii/S0304423819310362, (cited by 2021 Sep 24).
[https://doi.org/10.1016/j.scienta.2019.109150]
-
Palmer JD. (1985). Comparative organization of chloroplast genomes. Annual Reviews of Genetics. 19:325-354.
[https://doi.org/10.1146/annurev.ge.19.120185.001545]
-
Park HS, Jayakodi M, Lee SH, Jeon JH, Lee HO, Park JY, Moon BC, Kim CK, Wing RA, New master SG, Kim JY and Yang TJ. (2020). Mitochondrial plastid DNA can cause DNA barcoding paradox in plants. Scientific Reports. 10:1-12.
[https://doi.org/10.1038/s41598-020-63233-y]
-
Park SI, Hwangbo K, Gil JS, Chung H, Kim HB, Kim OT, Kim SC, Koo SC, Um Y and Lee Y. (2017). Determination of the origin of Angelica roots using Angelica gigas chloroplast based SSR markers. Korean Journal of Medicinal Crop Science. 25:361-366.
[https://doi.org/10.7783/KJMCS.2017.25.6.361]
-
Park YS, Park JY, Kang JH, Lee WH and Yang TJ. (2021). Diversity and authentication of Rubus accessions revealed by complete plastid genome and rDNA sequences. Mitochondrial DNA Part B. 6:1454-1459.
[https://doi.org/10.1080/23802359.2021.1911712]
-
Sang T, Crawford DJ and Stuessy TF. (1997). Chloroplast DNA phylogeny, reticulate evolution, and biogeography of Paeonia (Paeoniaceae). American journal of Botany. 84:1120-1136.
[https://doi.org/10.2307/2446155]
-
Sloan DB and Wu Z. (2014). History of plastid DNA insertions reveals weak deletion and at mutation biases in angiosperm mitochondrial genomes. Genome Biology and Evolution. 6:3210-3221.
[https://doi.org/10.1093/gbe/evu253]
-
Taberlet P, Gielly L, Pautou G and Bouvet J. (1991). Universal primers for amplification of three non-coding regions of chloroplast DNA. Plant Molecular Biology. 17:1105-1109.
[https://doi.org/10.1007/BF00037152]
- Tate JA and Simpson BB. (2003). Paraphyly of Tarasa(Malvaceae) and diverse origins of the polyploid species. Systematic Botany. 28:723-737.
-
Tillich M, L ehw ark P, P ellizzer T, Ulbricht-Jones E S, F ischer A, Bock R and Greiner S. (2017). GeSeq-versatile and accurate annotation of organelle genomes. Nucleic Acids Research. 45:w6-w11.
[https://doi.org/10.1093/nar/gkx391]
-
Tulio Jr AZ, Reese RN, Wyzgoski FJ, Rinaldi PL, Fu R, Scheerens JC and Miller AR. (2008). Cyanidin 3-rutinoside and cyanidin 3-xylosylrutinoside as primary phenolic antioxidants in black raspberry. Journal of Agricultural and Food Chemistry. 56:1880-1888.
[https://doi.org/10.1021/jf072313k]
-
Wicke S, Schneeweiss GM, dePamphilis CW, Müller KF and Quandt D. (2011). The evolution of the plastid chromosome in land plants: Gene content, gene order, gene function. Plant Molecular Biology. 76:273-297.
[https://doi.org/10.1007/s11103-011-9762-4]
-
Wolfe KH, Li WH and Sharp PM. (1987). Rates of nucleotide substitution vary greatly among plant mitochondrial, chloroplast, and nuclear DNAs. Proceeding of the National Academy of Sciences of the United States of America. 84:9054-9058.
[https://doi.org/10.1073/pnas.84.24.9054]