
Comparison of Major Compounds of Coffee Tree Leaf Extract by Varieties Cultivated in Korea
This is an open access article distributed under the terms of the Creative Commons Attribution Non-Commercial License (http://creativecommons.org/licenses/by-nc/3.0/) which permits unrestricted non-commercial use, distribution, and reproduction in any medium, provided the original work is properly cited.
Abstract
To identify suitable varieties of coffee for Korea, various varieties of coffee trees are being cultivated. In relation to this process, it is necessary to develop the analysis methods to confirm and compare the characteristics of each variety.
In this study, ethanol extracts of coffee tree leaves of four varieties, Coffea arabica var. bourbon (Bourbon), C. arabica var. maragogype (Maragogipe), C. arabica var. typica (Typica), and C. arabica var. geisha (Geisha), were targeted. The liquid chromatography (LC) profile of each extract was analyzed using a ultra-violet detector. Sixteen major peaks were selected from the LC profile analysis results, and mass spectrometry (MS) was performed using an LC-MS/MS system with the same separation conditions. The MS results confirmed that the major peaks were caffeoyl quinic acid, procyanidin, dicaffeoyl quinic acid, mangiferin, cinchonain I isomer, iriflophenone 3-C-glucoside, caffeine, and theobromine. The quantities of these major compounds were compared for each variety through additional multiple reaction monitoring mode MS analysis.
Differences in the major compound contents of the leaves of different domestic coffee tree varieties were confirmed, and LC-MS/MS analysis was found to be useful for the comparative analysis of compounds between varieties.
Keywords:
Coffea arabica, Coffee Leaves, Compound Profile, Liquid Chromatography, Mass Spectrometry, VarietiesINTRODUCTION
Coffee is a crop grown in tropical and subtropical climates called the Coffee Zone, located between latitudes 22 degrees north and latitude 26 degrees south. Since Korea is located at 33 to 43 degrees north latitude, the temperature is relatively cold compared to the existing coffee growing regions. Therefore, although production is difficult in Korea's natural environment, cultivation is gradually increasing using facility cultivation methods in Jeju, Jeonnam, and Gyeongnam areas (Moon et al., 2019; Kim, 2020).
The most representative coffee tree varieties worldwide are Coffea arabica, C. canephora, and C. liberica (Davis et al., 2006). Among them, the varieties belonging to C. arabica are known to be more suitable for the cultivation environment of Korea. In particular, C. arabica varieties suitable for high-altitude areas such as C. arabica var. typica (Typica) and C. arabica var. bourbon (Bourbon) are considered preferentially (WCR, 2019).
The value of coffee tree leaves is ignored or of little interest because of the value possessed by the coffee beans that are mainly used. However, it has been traditionally used for the treatment of various diseases or alleviation of symptoms, mainly in the major coffee growing regions (Ngamsuk et al., 2019). In addition, research on various compounds present in the leaves of coffee trees and studies to increase the utility are continuously being conducted (Chen et al., 2018; Chen et al., 2019; de Almeida et al., 2019; Monteiro et al., 2020; Cangeloni et al., 2022 Maxiselly et al., 2022).
Various studies on the compounds and activity of coffee beans are being conducted in Korea (Nam and Kang, 2015; Kim and Lee, 2018; Lee et al., 2018; Shin, 2019). In addition, some studies on the activity and compounds of coffee tree leaves grown in Korea were also conducted (Im and Doo, 2021a; Im and Doo, 2021b; Lee et al., 2022). Except for these few studies, there are still few studies on the physiological activity or compounds of coffee trees grown in Korea.
The compound profile of a plant varies depending on the plant cultivar, growing region, climate and vegetation stage along with the cultivation process (Cangeloni et al., 2022). As far as is known, coffee leaves contain numerous compounds such as caffeine, chlorogenic acid, and mangiferin (Campa et al., 2012; Chen et al., 2019; Mendes et al., 2019; Monteiro et al., 2020). Various compounds, including these active compounds, show different contents depending on the environment such as the variety or growing area. Therefore, it is necessary to continuously study the compound analysis of coffee trees grown in a new environment in Korea.
Compound profile analysis is a method used when it is not possible to specify a index compound or for quality control of herbal medicines. As an analysis performed without a standard compound, the difference between samples is confirmed by comparing the specific LC chromatogram pattern of each plant. The plant extract used for this profile analysis is mixed with at least hundreds of compounds. Therefore, even if the separation method is optimized, there is a limit in the general liquid chromatography (LC) system to completely separate all compounds present in the extract. In addition, it is practically difficult to prepare standards for chromatographic analysis for all compounds. Mass spectrometry (MS) is increasingly being used as an analysis equipment that can overcome these problems (Lee et al., 2022).
LC-MS/MS having a triple quadrupole structure is capable of multiple reaction monitoring (MRM) or selected reaction monitoring (SRM) mode analysis as well as scan and selected ion monitoring (SIM) mode analysis that can be performed in general LC-MS. MRM or SRM mode is an analysis that selectively detects a specific product ion generated from a specific molecular ion (Na et al., 2020). It is possible to minimize the influence of adjacent compounds in general LC analysis, and it is possible to analyze compounds with overlapping retention times (Im and Lee, 2020).
On the other hand, high-resolution MS such as time of flight (TOF) MS, which have superior qualitative analysis capability than tandem MS having such a triple quadrupole structure, are being used. However, the use of tandem MS is often considered first due to the initial cost or relative ease of operation. In particular, tandem MS can be effective in the search and quantitative analysis of compounds known through various existing studies.
In this study, the LC profiles of major compounds present in extracts of coffee tree leaf samples were compared to determine the differences between coffee tree varieties grown in Korea. For the coffee tree leaf sample, 4 types that are widely cultivated in the same area were selected. MS analysis in scan mode was performed on the major compound peaks identified in the LC profile analysis, and each compound was identified with reference to existing studies. In addition, by setting the conditions for MS analysis in MRM mode, the applicability of the method for rapid comparative analysis of major compounds in coffee leaves was confirmed.
MATERIALS AND METHODS
1. Plant materials
Coffee tree leaves of 4 varieties Coffea arabica var. bourbon (Bourbon), C. arabica var. maragogype (Maragogipe), C. arabica var. typica (Typica), and C. arabica var. geisha (Geisha) were collected in 2022 from those grown in Hwasun, Jeollanam-do (Fig. 1).
The age of each coffee tree variety was between 13 and 15 years, and only mature leaves free from pests and diseases were collected. The samples were dried at 50℃ for 48 hours. The dried sample was pulverized to a size of 0.25 ㎜ or less using a blender and used for extraction.
2. Preparation of extraction
Extraction was performed by mixing 200 ㎖ of ethanol with 10 g of a coffee tree leaf sample for each variety. Shaking extraction was performed at a speed of 180 rpm for 24 hours.
Extraction for each sample was performed three times separately and used for analysis. After extraction, it was filtered with a syringe filter (0.45 ㎛) and stored at 4℃ for use in the experiment.
3. LC profile analysis
LC-30A (Shimadzu, Kyoto, Japan) liquid chromatography and Kinetex C18 (2.1 ㎜ × 100 ㎜, 1.7 ㎛, Phenomenex, Torrance, CA, USA) column were used to analyze the LC profile of coffee tree leaf extract.
The sample injection volume was set at 1 ㎕, and the column oven was set at 40℃. 0.1% formic acid (A) and methanol (B) were used as mobile phases, and the flow rate was maintained at 0.3 ㎖/min. Gradient program for profile analysis: from 0.0 to 5.0 min 5% B (isocratic), from 5.0 to 25.0 min 5 - 40% B (linear), from 25.0 to 32.0 min 40 - 100% B (linear), From 32.0 to 38 min 100% B (isocratic), from 38.0 to 38.5 min 100 - 5% B (linear), from 38.5 to 45.0 min 5% B (isocratic).
The compounds for mass spectrometry were selected based on the 280 ㎚ analysis results showing the most diverse compound profile.
4. LC-MS/MS analysis of major compounds
LC-30A (Shimadzu Co., Kyoto, Japan) liquid chromatography and LCMS-8050 (Shimadzu Co., Kyoto, Japan) triple quadrupole mass spectroscopy were used for mass spectrometry of major compounds of coffee tree leaf extract.
The mobile phase and separation conditions used in the LC profile analysis were applied. An electro-spray ionization (ESI) device was used for ionization for mass spectrometry of major compounds. Scan mode analysis was performed in both positive and negative polarity, and multiple reaction monitoring (MRM) mode analysis conditions were set by applying polarity with excellent sensitivity or specificity for each compound. The collision-induced dissociation gas (argon) pressure was set 270 kPa. The nebulizing gas, the drying gas, and the heating gas flow were set to 3 ℓ/min, 10 ℓ/min, and 10 ℓ/min, respectively.
The mass spectra were scanned 100 to 1,200 m/z. Other MS conditions included interface temperature 300℃, desolvation line temperature 250℃, heat block temperature 350℃.
RESULTS AND DISCUSSION
1. LC profile of coffee tree leaf extract by varieties
In order to confirm the profile of various compounds present in the coffee tree leaf extract, LC analysis was performed under 200 ㎚ - 400 ㎚ UV detector conditions. The compound profiles of 4 coffee tree leaf extracts by varieties were compared based on the result chromatogram of 280 ㎚, in which the compound profile was varied and clearly identified among the detector wavelength conditions (Fig. 2).
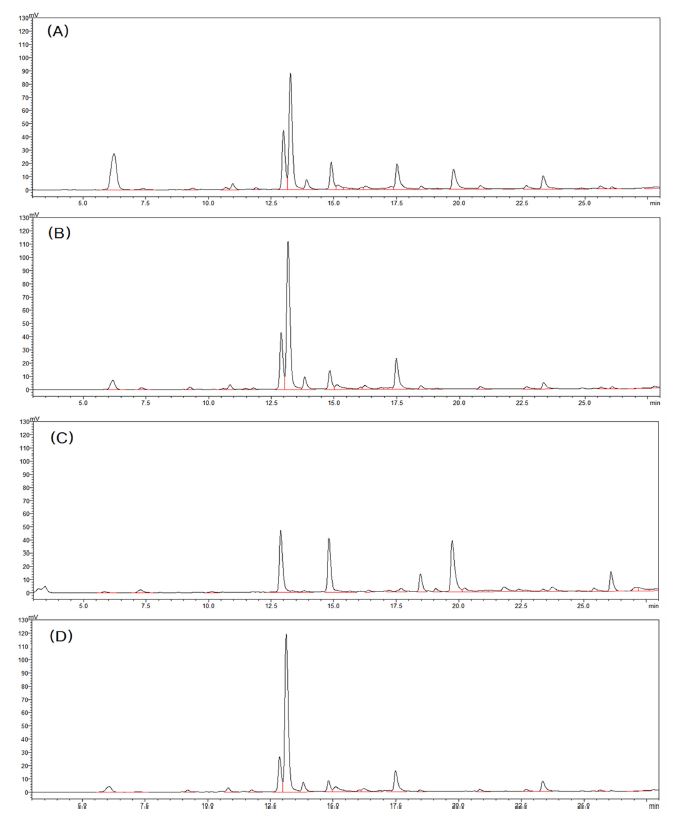
LC profile of coffee tree leaf extract by varieties.(A) Typica, (B) Bourbon, (C) Maragogipe, (D) Geisha.
In the analysis results, there were compound peaks that were common to all varieties, but also compounds with very low or no content were identified in some varieties. In particular, it was confirmed that the compound profile of Maragogipe variety (Fig. 2C) was significantly different from other varieties.
2. LC-MS analysis of major compound peaks
In the LC profile analysis result, the main peaks from 1 to 16 were selected from the Typica variety extract, which showed the most diverse compound peaks, and mass spectrometry of the peaks was performed (Fig. 3). For mass spectrometry, the same separation conditions as for LC profile analysis were applied, and the mass spectrometry results of each peak were reviewed based on the retention time of the LC profile analysis results.
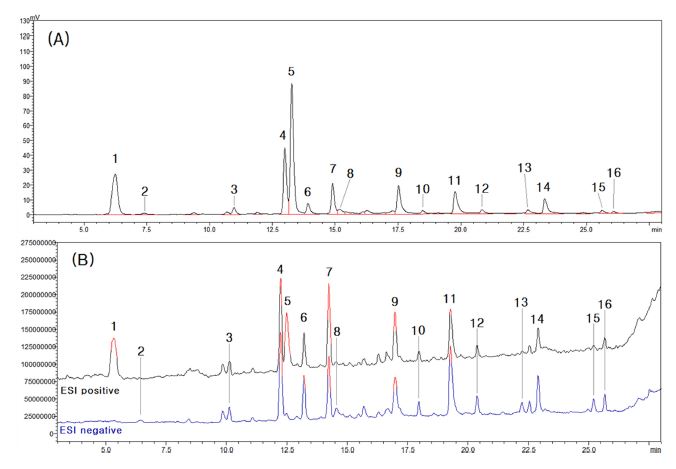
LC profile (A) and LC-MS total ion chromatogram (B) of extracts from coffee tree leaves of the Typica variety.Different superscripts (1 - 16) are major compounds.
In general, in a mass spectrometer based on an electrospray ionization (ESI) device, the molecular weight can be estimated by comparing [M+H]+, which is a molecular ion pattern in positive mode, and [M-H]-, a molecular ion pattern, shown in negative mode (Lee et al., 2022). On the other hand, the molecular ion pattern may appear selectively in the positive or negative mode due to the structural characteristics of the compound.
By comparing the main peak of the LC profile analysis result with the peak of the mass spectrometry result, the molecular weight of each peak was measured as shown in Table 1. 1 and 5 peak, which molecular ion was confirmed only in positive mode, were analyzed by MS2 in positive mode to confirm product ions. Except for peaks 1 and 5, the product ions were confirmed by MS2 analysis in negative mode.
In the MS spectra of peak 1 presented in Table 1, 181 m/z in the form of [M+H]+ was confirmed only in positive mode. Therefore, it was estimated as a compound with a molecular weight of 180, and product ions were confirmed in positive mode. The product ions generated from 181 m/z in positive mode were 163, 138, 67, and 42 m/z. This MS spectra was found to be in the form of theobromine by referring to the existing literature (Mendes et al., 2019).
Peak 2 was confirmed to be a compound with a molecular weight of 354 as 353 m/z in the form of [M-H]- in the negative mode and 355 m/z in the form of [M+H]+ in the positive mode. The product ions generated from 353 m/z in negative mode were 191, 179, and 135 m/z. This MS spectra was found to be a typical form of caffeoyl quinic acid-based compounds by referring to the existing literature (Bajko et al., 2016). The MS spectra of peak 4 also showed MS and MS2 spectra of a pattern similar to that of peak 2, so it could be estimated that it was a caffeoyl quinic acid-based compounds. By reviewing previous research reports, it was confirmed that peaks 2 and 4 were 5-caffeoyl quinic acid and 3-caffeoyl quinic acid, respectively (Zhang et al., 2015; Chen et al., 2018; Kim et al., 2020; Lee et al., 2022). Caffeoyl quinic acid is a major compound of coffee and has been reported as a compound with antioxidant activity in various studies (Monteiro et al., 2020; Lee et al., 2022).
Peak 3 was confirmed to be a compound with a molecular weight of 408 as 407 m/z in the form of [M-H]- in the negative mode and 409 m/z in the form of [M+H]+ in the positive mode. The product ions generated from 407 m/z in negative mode were 317, 287, 245, and 193 m/z. It was confirmed that iriflophenone 3-C-glucoside was compared with the existing literature related to the compound of coffee tree leaves (Cangeloni et al., 2022).
In the MS spectra of peak 5, 195 m/z in the form of [M+H]+ was confirmed only in positive mode. Therefore, it was estimated as a compound with a molecular weight of 194, and product ions were confirmed in positive mode. The product ions generated from 195 m/z in positive mode were 138, 123, 110, and 83 m/z. This MS spectra was found to be a typical form of caffeine by referring to the existing literature (Mendes et al., 2019).
In the MS spectra of peaks 6 and 12, 577 m/z in the form of [M-H]- in the negative mode and 579 m/z in the form of [M+H]+ in the positive mode were confirmed, so it was estimated as a compound with a molecular weight of 578. The product ions generated from 577 m/z in negative mode were 425, 407, 289, and 125 m/z. This MS spectra is a form that appears in the dimer structure of catechin or epicatechin. Compared with the existing literature on mass spectrometry studies of procyanidin compounds, it was confirmed that it was a B-type procyanidin dimer (Gu et al., 2003; Bakhytkyzy et al., 2018; Rue et al., 2018; Cangeloni et al., 2022). Procyanidin exhibits strong antioxidant activity of catechin or epicatechin, which constitutes the structure (Murakami et al., 2006; Calderón et al., 2009).
Peak 7 was confirmed to be a compound with a molecular weight of 290 as 289 m/z in the form of [M-H]- in the negative mode and 291 m/z in the form of [M+H]+ in the positive mode. The product ions generated from 289 m/z in negative mode were 245, 203, 123, and 109 m/z. This MS spectra was found to be a form that appears in the structure of catechin or epicatechin, and compared with the existing literature on mass spectrometry studies on catechin compounds, epicatechin was confirmed (Murakami et al., 2006; Calderón et al., 2009; Nemes et al., 2018).
Peak 8 was confirmed to be a compound with a molecular weight of 864 as 863 m/z in the form of [M-H]- in the negative mode and 865 m/z in the form of [M+H]+ in the positive mode. The product ions generated from 863 m/z in negative mode were 711, 573, 451, 411, and 289 m/z. Such MS spectra appear in structures derived from trimer forms of catechin or epicatechin. Compared with the existing literature on mass spectrometry of procyanidin compounds, it was confirmed that it was cinnamtannin B-1 (Rue et al., 2018; Rush et al., 2018; Lee et al., 2022). Cinnamtannin B-1 has been reported to have high antioxidant activity (Kim et al., 2016; Woo et al., 2017; Lee et al., 2022).
Peak 9 was confirmed to be a compound with a molecular weight of 422 as 421 m/z in the form of [M-H]- in the negative mode and 423 m/z in the form of [M+H]+ in the positive mode. The product ions generated from 421 m/z in negative mode were 331, 301, 271, and 259 m/z. This MS spectra was found to be a form of mangiferin present in coffee leaves (Campa et al., 2012; Chen et al., 2019; Ngamsuk et al., 2019; Monteiro et al., 2020). Mangiferin is a compound that has been reported for anticancer-related activity as well as antioxidant activity (Lee et al., 2018; Morozkina et al., 2021)
In the MS spectra of peaks 10 and 16, 451 m/z in the form of [M-H]- in the negative mode and 453 m/z in the form of [M+H]+ in the positive mode were confirmed, so it was estimated as a compound with a molecular weight of 452. The product ions generated from 451 m/z in negative mode were 341, 217, 189, and 177 m/z. It was confirmed that it is a cinchonain I isomer by comparing the MS spectra and the existing literature related to the study of coffee leaves (Cangeloni et al., 2022). Cinchonain I isomer is known to have antidiabetic, antioxidant and hepatoprotective effects (Gomes et al., 2017; Sobeh et al., 2017).
Peak 11 was confirmed to be a compound with a molecular weight of 576 as 575 m/z in the form of [M-H]- in the negative mode and 577 m/z in the form of [M+H]+ in the positive mode. The product ions generated from 575 m/z in negative mode were 449, 423, 289, and 285 m/z. This MS spectra is confirmed in the form shown in the A-type procyanidin dimer (Bakhytkyzy et al., 2018).
In the MS spectra of peaks 13, 14 and 15, 515 m/z in the form of [M-H]- in the negative mode and 517 m/z in the form of [M+H]+ in the positive mode were confirmed, so it was estimated as a compound with a molecular weight of 516. The product ions generated from 517 m/z in negative mode were 353, 335, 191, and 179 m/z. Such MS spectra are shown in compounds having a dicaffeoyl quinic acid structure in which two caffeoyl groups and quinic acid are combined (Clifford et al., 2005). By reviewing existing studies on the components of coffee leaves, it was confirmed that peaks 13, 14, and 15 were 3,4-dicaffeoyl quinic acid, 3,5-dicaffeoyl quinic acid, and 4,5-dicaffeoyl quinic acid, respectively (Campa et al., 2012; Chen et al., 2018; Monteiro et al., 2020). It has been reported that three types of dicaffeoyl quinic acid have anti-inflammatory activity as well as antioxidant activity (Chen et al., 2019; Kim et al., 2020).
3. Multiple reaction monitoring condition setting
Mass spectrometry in multiple reaction monitoring (MRM) mode selectively detects only specific ions generated from specific molecular ion. MRM mode analysis is useful for the analysis of samples in which hundreds of compounds are mixed, such as plant extracts (Na et al., 2020; Im and Lee, 2020).
Based on the results of LC-MS analysis of the major peaks presented in Table 1, molecule ions and product ions were selected. For qualitative and quantitative analysis, 2 MRM conditions were set for each compound as shown in Table 2.
In order to confirm the usefulness of the established MRM mode analysis method, comparative analysis was performed on the ethanol extract of coffee tree leaves by varieties. In the chromatogram of the MRM mode presented in Figure 4, the difference in the content of each compound was clearly confirmed for each extract. Since the detection of compounds other than the compounds to be analyzed was excluded, a simple and clear comparison was possible compared to the chromatogram using the ultra violet (UV) detector of general LC analysis.
4. Comparative analysis of extracts by varieties
Table 3 presents the results of comparative analysis of major components of coffee tree leaf extracts by varieties. Relative content results were calculated by comparing the peak areas of each compound for each varieties based on the peak area of the Typica variety extract.
Based on the content of the Typica variety, procyanidin dimer type A and theobromine in the extract of the Bourbon variety showed low relative content of 0.71% and 26.5%, respectively. In Geisha variety, 6 compounds showed a big difference of more than 50%. Similar to the Bourbon variety, procyanidin dimer type A was very low at 1.14%, and theobromine also showed a relatively low content at 19.98%. In addition, epicatechin, 4,5-dicaffeoyl quinic acid, cinchonain I isomer 1 and 2 showed a low relative content of less than 50%.
Compared to the Typica variety, the Maragogipe variety showed the most different compound relative content pattern. Specifically, theobromine and iriflophenone 3-C-glucoside were not detected in the extract of Maragogipe variety. Caffeine and mangiferin, which appeared in similar contents in extracts of other varieties, were confirmed only at trace levels. In addition, cinnamtannin B-1, 3,4-dicaffeoyl quinic acid, 3,5-dicaffeoyl quinic acid, 4,5-dicaffeoyl quinic acid, procyanidin dimer B-type 1 and 2 showed a low relative content of less than 50%. On the other hand, the content of cinchonain I isomer 1 and 2 were high around 700%, and epicatechin, procyanidin dimer A-type, and 5-caffeoyl quinic acid were found to be high at 174 - 220%.
In Korea, the cultivation of coffee trees is increasing by using the facility cultivation method. Therefore, various studies are needed to develop coffee-related products. The major compounds identified in coffee leaves grown in Korea through this study are 16 compounds, including caffeine and 3-caffeoylquinic acid. Most of the identified main componunds are compounds that exhibit physiological activities such as antioxidant activity (Calderón et al., 2009; Kim et al., 2016; Gomes et al., 2017; Sobeh et al., 2017; Woo et al., 2017; Lee et al., 2018; Chen et al., 2019; Kim et al., 2020; Morozkina et al., 2021; Lee et al., 2022).
By setting the MRM mode analysis conditions, the main compound analysis method of the coffee tree leaf extract using LC-MS/MS was established. The usefulness of the MRM mode analysis method was confirmed by performing comparative analysis of coffee tree leaf extracts by varieties. There were differences in some compounds depending on the variety, and the Maragogipe variety showed a significantly different compound content pattern compared to other varieties. In addition, differences in compounds occur in plants depending on conditions such as varieties, cultivation regions, and harvesting times. Therefore, the analysis method for these compounds will also need to be continuously improved.
These results can be used as basic data for domestic coffee tree cultivation related varieties and characteristics by production area, product development and quality control in related industries such as food, cosmetics, and pharmaceuticals.
References
-
Bajko E, Kalinowska M, Borowski P, Siergiejczyk L and Lewandowski W. (2016). 5-O-Caffeoylquinic acid: a spectroscopic study and biological screening for antimicrobial activity. LWT-Food Science and Technology. 65:471-479.
[https://doi.org/10.1016/j.lwt.2015.08.024]
-
Bakhytkyzy I, Nuñez O and Saurina J. (2018). Determination of flavanols by liquid chromatography with fluorescence detection. Application to the characterization of cranberry-based pharmaceuticals through profiling and fingerprinting approaches. Journal of Pharmaceutical and Biomedical Analysis. 156:206-213.
[https://doi.org/10.1016/j.jpba.2018.04.031]
-
Calderón AI, Wright BJ, Hurst WJ and van Breemen RB. (2009). Screening antioxidants using LC-MS: Case study with cocoa. Journal of Agricultural and Food Chemistry. 57:5693-5699.
[https://doi.org/10.1021/jf9014203]
-
Campa C, Mondolot L, Rakotondravao A, Bidel LPR, Gargadennec A, Couturon E, La Fisca P, Rakotomalala J, Jay-Allemand C and Davis AP. (2012). A survey of mangiferin and hydroxycinnamic acid ester accumulation in coffee(Coffea) leaves: Biological implications and uses. Annals of botany. 110:595-613.
[https://doi.org/10.1093/aob/mcs119]
-
Cangeloni L, Bonechi C, Leone G, Consumi M, Andreassi M, Magnani A, Rossi C and Tamasi G. (2022). Characterization of extracts of coffee leaves(Coffea arabica L.) by spectroscopic and chromatographic/spectrometric techniques. Foods. 11:2495. https://www.mdpi.com/2304-8158/11/16/2495, (cited by 2022 Aug 18).
[https://doi.org/10.3390/foods11162495]
-
Chen X, Kitts DD, Ji D and Ding J. (2019). Free radical scavenging activities of phytochemical mixtures and aqueous methanolic extracts recovered from processed coffee leaves. International Journal of Food Science and Technology. 54:2872-2879.
[https://doi.org/10.1111/ijfs.14099]
-
Chen X, Ma Z and Kitts DD. (2018). Effects of processing method and age of leaves on phytochemical profiles and bioactivity of coffee leaves. Food Chemistry. 249:143-153.
[https://doi.org/10.1016/j.foodchem.2017.12.073]
-
Clifford MN, Knight S and Kuhnert N. (2005). Discriminating between the six isomers of dicaffeoylquinic acid by LC-MSn. Journal of Agricultural and Food Chemistry. 53:3821-3832.
[https://doi.org/10.1021/jf050046h]
-
Davis AP, Govaerts R, Bridson DM and Stoffelen P. (2006). An annotated taxonomic of the genus Coffea(Robiaceae). Botanical Journal of the Linnean Society. 152:465-512.
[https://doi.org/10.1111/j.1095-8339.2006.00584.x]
-
de Almeida RF, Trevisan MTS, Thomaziello RA, Breuer A, Klika KD, Ulrich CM and Owen RW. (2019). Nutraceutical compounds: Echinoids, flavonoids, xanthones and caffeine identified and quantitated in the leaves of Coffea arabica trees from three regions of Brazil. Food Research International. 115:493-503.
[https://doi.org/10.1016/j.foodres.2018.10.006]
-
Gomes RM, de Paulo LF, Bonato Panizzon CPN, Neves CQ, Cordeiro BC, Zanoni JN, Francisco FA, Piovan S, de Freitas Mathias PC, Longhini R, de Mello JCP, de Oliveira JC, Pedrino GR, da Silva Reis AA, Cecchini AL and Marçal Natali MR. (2017). Anti-diabetic effects of the ethylacetate fraction of Trichilia catigua in streptozotocin-induced type 1 diabetic rats. Cellular Physiology and Biochemistry. 42:1087-1097.
[https://doi.org/10.1159/000478761]
-
Gu L, Kelm MA, Hammerstone JF, Beecher G, Holden J, Haytowitz D and Prior RL. (2003). Screening of foods containing proanthocyanidins and their structural characterization using LC-MS/MS and thiolytic degradation. Journal of Agricultural and Food Chemistry. 51:7513-7521.
[https://doi.org/10.1021/jf034815d]
- Im DY and Doo YT. (2021a). Analysis of characteristics by extraction conditions for product development using coffee tree leaves. Journal of Product Research. 39:53-58.
-
Im DY and Doo YT. (2021b). A study on the physiological activity of coffee tree leaf extract as a cosmetic material. Journal of Business Convergence. 6:63-68.
[https://doi.org/10.31152/JB.2021.08.6.3.63]
- Im DY and Lee KI. (2020). LC-MS/MS screening method for radical scavenging active compounds in extracts of Ulmus pumila cortex. Journal of Life Science. 30:956-964.
- Kim IH and Lee JH. (2018). Antibacterial and whitening activities of Coffea arabica ethanol extract. Korean Chemical Engineering Research. 56:245-251.
- Kim IH. (2020). Study on the characteristics of green and roasted coffee beans cultivated in Korea and foreign countries. Ph. D. Thesis. Sejong University. p.1-3.
- Kim MS, Woo KW, Lee KH, Lee HJ, Lee SY, Kang BM, Jeon BH, Cho JH and Cho HW. (2016). Isolation and quantitative analysis of cinnamtannin B-1 from Osmunda japonica Thunb. Korean Journal of Pharmacognosy. 47:232-236.
-
Kim SM, Kim AY and Lee KI. (2020). Nitric oxide production inhibitory effects of three caffeoylquinic acids isolated from hot water extract of Eriobotrya japonica L. leaves. Korean Journal of Medicinal Crop Science. 28:245-253.
[https://doi.org/10.7783/KJMCS.2020.28.4.245]
- Lee KE, Son SH and Kang SG. (2018). The coffee sliver skin extracts from coffee beans exhibited cosmetic properties with antioxiant activity and inhibitory effects for elastase, collagenase and tyrosinase. Journal of the Society of Cosmetic Scientists of Korea. 44:39-48.
-
Lee KI, Pyo BS, Choi CH and Cha SW. (2022). Radical scavenging active compound screening analysis in extract of Coffea arabica L. leaves. Korean Journal of Medicinal Crop Science. 30:264-277.
[https://doi.org/10.7783/KJMCS.2022.30.4.264]
-
Maxiselly Y, Anusornwanit P, Rugkong A, Chiarawipa R and Chanjula P. (2022). Morpho-physiological traits, phytochemical composition, and antioxidant activity of Canephora coffee leaves at various stages. International Journal of Plant Biology. 13:106-114. https://www.mdpi.com/2037-0164/13/2/11, (cited by 2022 May 23)
[https://doi.org/10.3390/ijpb13020011]
-
Mendes VM, Coelho M, Tomé AR, Cunha RA, and Manadas B. (2019). Validation of an LC-MS/MS method for the quantification of caffeine and theobromine using non-matched matrix calibration curve. Molecules. 24:2863. https://www.mdpi.com/1420-3049/24/16/2863, (cited by 2022 Aug 7).
[https://doi.org/10.3390/molecules24162863]
-
Monteiro Â, Colomban S, Azinheira H, Guerra-Guimarães L, Silva MD, Navarini L and Resmini M. (2020). Dietary antioxidants in coffee leaves: impact of botanical origin and maturity on chlorogenic acids and xanthones. Antioxidants. 9:6. https://www.mdpi.com/2076-3921/9/1/6, (cited by 2022 Dec 20).
[https://doi.org/10.3390/antiox9010006]
-
Moon SY, Baek SY and Kim MR. (2019). Determination of aroma profiles of coffee cultivated in Goheung, Korea by gas chromatography-ion mobility spectrometry. Korean Journal of Food Preservation. 26:576-585.
[https://doi.org/10.11002/kjfp.2019.26.5.576]
-
Morozkina SN, Vu THN, Generalova YE, Snetkov PP and Uspenskaya MV. (2021). Mangiferin as new potential anti-cancer agent and mangiferin-integrated polymer systems-a novel research direction. Biomolecules. 11:79. https://www.mdpi.com/2218-273X/11/1/79, (cited by 2022 Jan 9).
[https://doi.org/10.3390/biom11010079]
- Murakami I, Nakamura T, Ishibashi Y, Shibuya R, Ayano E, Morita-Murase Y, Nagata Y and Kanazawa H. (2006). Simultaneous determination of catechins and procyanidins in bottled tea drinks by LC/MS. Chromatography. 27:27-33.
-
Na ES, Kim SS, Hong SS, Kim KJ, Lee YJ, Lee BC and Lee SK. (2020). Development of multi-residue analytical method for 261 pesticides in herbal medicines using GC-MS/MS and LC-MS/MS. The Korean Journal of Environmental Agriculture. 39:142-169.
[https://doi.org/10.5338/KJEA.2020.39.2.19]
-
Nam S and Kang S. (2015). Changes of biochemical components and physiological activities of coffee beans according to different roasting conditions. Korean Journal of Food Preservation. 22:182-189.
[https://doi.org/10.11002/kjfp.2015.22.2.182]
-
Nemes A, Szőllősi E, Stündl L, Biró A, Homoki J, Szarvas M, Balogh P, Cziáky Z and Remenyik J. (2018). Determination of flavonoid and proanthocyanidin profile of Hungarian sour cherry. Molecules. 23:3278. https://www.mdpi.com/1420-3049/23/12/3278, (cited by 2022 Aug 11).
[https://doi.org/10.3390/molecules23123278]
-
Ngamsuk S, Huang TC and Hsu JL. (2019). Determination of phenolic compounds, procyanidins, and antioxidant activity in processed Coffea arabica L. leaves. Foods. 8:389. https://www.mdpi.com/2304-8158/8/9/389, (cited by 2022 Aug 4).
[https://doi.org/10.3390/foods8090389]
-
Rue EA, Rush MD and van Breemen RB. (2018). Procyanidins: A comprehensive review encompassing structure elucidation via mass spectrometry. Phytochemistry Reviews. 17:1-16.
[https://doi.org/10.1007/s11101-017-9507-3]
-
Rush MD, Rue EA, Wong A, Kowalski P, Glinski JA and van Breemen RB. (2018). Rapid determination of procyanidins using MALDI-ToF/ToF mass spectrometry. Journal of Agricultural and Food Chemistry. 66:11355-11361.
[https://doi.org/10.1021/acs.jafc.8b04258]
- Shin HK. (2019). Analysis of antioxidant components in coffee making process using washed coffee and natural coffee. The Korean Journal of Food and Nutrition. 32:312-320.
-
Sobeh M, Mahmoud MF, Sabry OM, Adel R, Dmirieh M, El-Shazly AM and Wink M. (2017). HPLC-PDA-MS/MS characterization of bioactive secondary metabolites from Turraea fischeri bark extract and its antioxidant and hepatoprotective activities in vivo. Molecules. 22:2089. https://www.mdpi.com/1420-3049/22/12/2089, (cited by 2022 Aug 29).
[https://doi.org/10.3390/molecules22122089]
-
Woo KW, Jung KJ, Lee HJ, Kim TM, Kim MS, Jung HK, An BK, Ham SH, Jeon BH and Hyun Woo Cho HW. (2017). Phytochemical constituents from the rhizomes of Osmunda japonica Thunb and their anti-oxidant activity. Natural Product Sciences. 23:217-221.
[https://doi.org/10.20307/nps.2017.23.3.217]
- World Coffee Research(WCR). (2019). Arabica coffee varieties. World Coffee Research. Portland. Oregon, USA. p.1-25.
-
Zhang H, Xi W, Yang Y, Zhou X, Liu X, Yin S, Zhang J and Zhou Z. (2015). An on-line HPLC-FRSD system for rapid evaluation of the total antioxidant capacity of Citrus fruits. Food Chemistry. 172:622-629.
[https://doi.org/10.1016/j.foodchem.2014.09.121]