
Changes in Amino acids, Minerals and Volatile Composition of Wild Garlicaccording to Forest Cultivation Environment
This is an open access article distributed under the terms of the Creative Commons Attribution Non-Commercial License (http://creativecommons.org/licenses/by-nc/3.0/) which permits unrestricted non-commercial use, distribution, and reproduction in any medium, provided the original work is properly cited.
Abstract
Wild garlic (Allium victorialis var. platyphyllum Makino) is a useful wild vegetable with excellent texture and good profitability. It is produced in an environmentally friendly manner through forest cultivation. At present optimal cultivation conditions remain to be identified. We investigated the impact of inorganic nutrients available to wild garlic grown in various environments on free amino acid and volatile compounds content.
While minerals available to wild garlic grown in a given cultivation environment were observed to vary with plot and cultivation year, no trend was identified. Free amino acid content varied significantly among cultivation plots, and was especially high in Chamaecyparis obtusa (Siebold and Zucc.) Endl. (CO) forest. Wild garlic free amino acid content increased rapidly during storage in its year after cultivation. Nineteen to twenty types of volatile compounds were detected. The main volatile compounds were sulfur compounds such as dimethyl trisulfide, disulfide, and methyl 2-propenyl. Little variation in total content by cultivation region was noted; however, differences in specific components were found.
These results can inform effective production of high-quality wild garlic by aiding in selection of an appropriate vegetation community for wild garlic cultivation.
Keywords:
Wild Garlic, Forestry Cultivation, Amino Acids, Inorganic Ingredients, Volatile CompoundsINTRODUCTION
Wild garlic (Allium victorialis var. platyphyllum Makino) is a herbaceous perennial plant in the lily family. It grows naturally in the forests of central region in Korea, including Mt. Jiri, Mt. Seorak, Mt. Odae, and Ulleung Island. The entire plant has a strong garlic scent, spicy taste, and non-poisonous.
Wild garlic is very similar to garlic in its smell and medicinal use (Chang et al., 2011). It belongs to the same genus, Allium, as garlic, but its form is entirely different from that of garlic.
In Korea, when inflorescences grow among fallen leaves in March to April after winter, young leaves and shoots are collected in May and used for food (Kwon et al., 2010).
Wild garlic, like garlic, has long been known as a traditional stamina food (Chang et al., 2011). Additionally, in Korea, it has been used as a folk remedy for hypertension, arteriosclerosis, gastritis, constipation, abdominal pain, forgetfulness, and insomnia (Kim et al., 2010). Wild garlic is edible for its tender leaves and the whole plant, including its stems and flowers. It has a unique taste and aroma and is rich in various minerals and vitamins, so the demand for it as a high-quality wild vegetable is increasing (Kim et al., 2012).
Recently, as the mechanism for promoting vitamin B absorption in the human body and the existence of anti-thrombotic substances were discovered, it has been attracting attention as a functional food and pharmaceutical ingredient (Lee, 1985; Choi et al., 2005).
The secondary metabolite content of medicinal plants is influenced by environmental factors, including climate and ecological issues (Cragg and Newman, 2013). Environmental conditions such as temperature changes, light intensity, high carbon dioxide, ultraviolet rays, ozone, drought, salinity, and flooding have a significant impact on the growth and development of medicinal plants and the synthesis of secondary metabolites (Verpoorte and Memelink, 2002; Imadi et al., 2015; Gupta et al., 2019; Li et al., 2020).
Therefore, plants for producing biomaterials with high content of biologically active substances and high productivity must be grown under optimal environmental conditions to ensure production efficiency (Kim et al., 2015).
Edible plants growing in the wild may face the risk of extinction due to excessive collection in their native habitat and continued increase in demand, and indiscriminate collection may damage the forest ecosystem and forests, resulting in ecosystem disturbance (Choi et al., 1993; Kim and Um, 1997). It may cause damage to resources.
Therefore, to produce sustainable non-timber forest products while minimizing these problems, it is necessary to develop production technologies for rational and environmentally friendly forestry cultivation (Kim et al., 2015).
Forest cultivation is the cultivation of useful plants in forests with an environment similar to their native habitat. It provides suitable shade and site conditions for marketable non-timber forest products and diversify income opportunities to forest farmers (Gold et al., 2018). Compared to general cultivation methods, growth is slower, and yields are lower, but the texture and flavor are much better in the forest cultivation (NiFoS, 2014). Moreover, forest cultivation requires lower production cost compared to agricultural cultures.
However, wild garlic is difficult to propagate, and forestry cultivation technology needs to be established appropriately (NiFoS, 2014). Selecting optimal cultivation conditions is very important for the production of high-quality wild garlic through forest cultivation, which has potential for use in functional foods or small-scale and high-value markets. Accordingly, this study was conducted to identify the optimal site for forestry cultivation by investigating changes in amino acids, inorganic components, and volatile compounds depending on the vegetation environment of the forestry cultivation area.
MATERIALS AND METHODS
1. Overview of wild garlic samples and test strips
The wild garlic used in this experiment was from broad-leaf forests (Acer palmatum, Aesculus turbinata) and coniferous forests (Chamaecyparis obtusa, Pinus koraiensis) from Gyeongsang National University Jirisan Experimental Forest (located in Sancheong-gun, Gyeongsangnam-do).
Bare ground, not a forest, was used as a negative control for forestry cultivation (Fig. 1). The test sites were set with five different sites at 10 m × 10 m each: C. obtusa forest (CO), A. palmatum forest (AP), A. turbinata forest (AT), and the bare ground located in one place and P. koraiensis forest (PK), located 2.3 ㎞ away from the main test site.
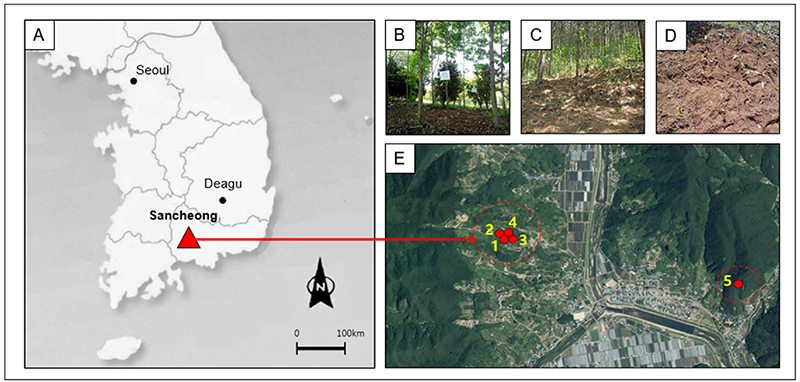
Five test strips of this study. A and E; experimental site locations in Korea, B; A. turbinata forest, C; P. koraiensis forest, and D; Bare ground. 1; A. turbinata, 2; C. obtusa, 3; Bare ground, 4; A. palmatum, 5; P. koraiensis.
The average elevation of the wild garlic plantation was 168 m (140 - 223 m), 77% (30% - 95%) of the tree layer, 16% (5% - 30%) of the shrub layer, and 35% (10% - 90%) of the herbaceous layer (Table 1). These sites were selected to investigate the effect of dominance species and canopy cover on the composition and amount of chemical compounds produced by wild garlic.
The soil chemical properties of experimental sites were analyzed according to National Institute of Agricultural Science (NIAS, 2010). Soil pH was highest in CO and AP soil, and lowest in PK soil (Table 2).
The amount of organic matters was highest in PK soil, and lowest in CO soil. In CO soil, total N content and cation exchange capacity were lower than those of other forests. AP soil exhibited high total N and available P. BG soil had high level of available P, whereas its cation exchange capacity was lower than that of other forests.
Wild garlic seeds were collected from the Jirisan Experimental Forest of Gyeongsang National University in July 2017. They were sown in forest soil under a greenhouse and grown for two years. In May 2020, each of the 200 seedlings were planted in five test sites. Before planting, all above-ground parts of the wild garlic in each site were collected, washed, and used for component analysis.
The analysis was also conducted in May of the following year to investigate the change in the chemical compound content of wild garlic. All parts above ground including leaves were freeze-dried at -72℃, ground, prepared into a uniform powder of 80 mesh, and then stored frozen at -20℃ for use in analysis.
2. Analysis of minerals
The analysis of mineral components was performed by pretreatment using the dry decomposition method of the Korea Food and Drug Administration (MFDS, 2022).
1 g of each of the above-ground freeze-dried wild garlic was placed in a 250 ㎖ flask, and 25 ㎖ of wet decomposition solution (HNO3 : H2SO4 : HClO4 = 10 : 1 : 4) was added and boiled in a heating mantle for over 3 hours. The residue and liquid were separated using Whatman filter paper, and the liquid was finally adjusted to 100 ㎖ using distilled water.
The treated samples were analyzed using an inductively coupled plasma spectrometer (ICP spectrometer, OPTIMA 4300DV, Perkin Elmer, Massachusetts, USA).
3. Free amino acid determination
Free amino acid analysis was performed by slightly modifying the method of Jeong et al. (2006).
To 3 g of wild garlic sample, 100 ㎖ of 75% Et-OH was added to homogenize, extracted by shaking at 50℃ for 2 hours, cooled to 4℃, and centrifuged at 6,000 rpm for 10 minutes. Then, The supernatant was concentrated using a rotary vacuum concentrator and adjusted to 25 ㎖ with distilled water. Then, sulfosalicylic acid (50 ㎎ per 1 ㎖ of sample) was added, allowed to stand for 1 hour, and centrifuged at 8,000 rpm for 10 minutes.
Then, the supernatant was filtered through a 0.22 ㎛ membrane filter and analyzed with an amino acid analyzer (LKB 4150 Alpha, LKB Ltd., Cambridge, England).
4. Volatile compound analysis
0.5 g of wild garlic sample was mixed with 1.5 ㎖ of tertiary distilled water and analyzed using a GC/MS (Clarus 600/Claus 600T, Perkin Elmer, Massachusetts, USA) equipped with headspace.
The initial oven temperature was 40℃ and maintained for 3 minutes, raised at 5℃/min to 40℃, raised at 10℃/min to 280℃, and maintained at 300℃ for 3 minutes. Helium was used as the carrier gas, the ion source temperature was 230℃, and the injector temperature was 250℃. Headspace conditions were oven temperature 120℃, transfer line temperature 125℃, and compound identification of wild garlic samples was confirmed by comparing NIST05S and Wiley7 Library.
5. Statistical analysis
Analysis of mineral, amino acid, and volatile compound was repeated at least three times and expressed as mean and standard deviation, and the results were analyzed by one-way ANOVA using the SPSS 20.0 software package (Statistical Package for Social Sciences, SPSS Inc., Chicago, IL, USA).
Means of regional ingredient compounds were compared at a 5% significance level using Duncan's Multiple Range Test (DMRT) comparisons. Means of the ingredient compounds before and after planting were compared using a t-test, also at a 5% significance level.
In addition, Pearson’s correlation analysis was conducted to investigate the effect of soil chemical properties on the content of minerals, amino acids, and volatile compounds of wild garlic.
RESULTS
1. Changes in mineral contents in forestry cultivation sites
The mineral composition of wild garlic leaves planted in five sites showed slight differences (Table 3). The most abundant mineral was potassium, followed by calcium and phosphorus. Among the five sites, the one with the most mineral content was CO forest, followed by PK and AT forest. The mineral composition changed after planting wild garlic (Table 3).
The mineral content was compared between before planting and after planting to investigate the change in the content influenced by forestry cultivation sites (Fig. 2). After planting wild garlic, the content of minerals in the above-ground increased at five experimental sites. In the wild garlic cultivated in CO forest, K, Mn, and P content increased.
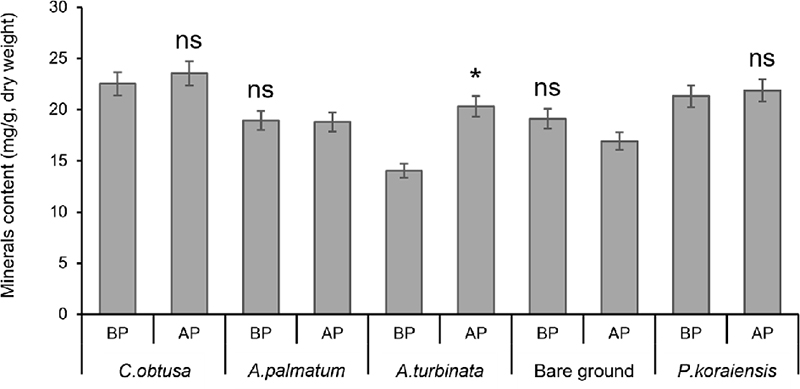
Mineral content before and after planting wild garlic at five planting sites. Bars indicate means of 3 replications and error bars indicate standard deviations. BP; before planting, AP: after planting, ns: not significantly different according to t-test.Signifcantly different according to t-test (*p < 0.05).
K, which showed the highest content among the mineral components, decreased in BG forest, while its content increased in CO, AP, AT, and PK forests (Fig. 2). Fe content increased in all of the cultivation areas except CO forest and BG, and Al content decreased only in CO forest cultivation areas. In particular, the P component increased in all cultivation environments.
2. Changes in free amino acid content in forest cultivation sites
The free amino acid content of wild garlic differed depending on the cultivation environment (Table 4).

The free amino acid content of wild garlic cultivated in different experimental sites (㎎/g, dry weight).
The most abundant free amino acid was urea, followed by L-asparagine, L-alanine, and L-leucine. Hydroxy-L-proline was detected in CO forest with high content, but it was not detected in the other sites. It was previously detected in AT and PK forest before planting, but not detected after planting. In AP forest, urea (5.03 ㎎/g), L-aspartic acid (2.10 ㎎/g), L-asparagine (3.84 ㎎/g), L-alanine (3.81 ㎎/g) and L-valine (3.09 ㎎/g) content increased, and amino acid content increased in wild garlic planted in AT forest included urea (13.14 ㎎/g), L-aspartic acid (6.03 ㎎/g), L-threonine (3.15 ㎎/g), and L-asparagine (2.38 ㎎/g). In BG, the content of urea (12.62 ㎎/g), L-threonine (3.65 ㎎/g), L-asparagine (3.12 ㎎/g), and L-glutamic acid (3.06 ㎎/g) increased. The urea, L-threonine, L-asparagine, and L-glutamic acid contents of wild garlic grown in PK forest were commonly increased.
The content of each free amino acid changed after wild garlic was planted in five different types of forest. In AP, BG, and PK forest, the content of over 24 kinds of free amino acid increased after planting: the content of 28, 24, 24 kinds of free amino acid increased in each forest. However, the content of only 14 kinds of free amino acid increased in CO forest. In AT forest, the number of free amino acid of which the content increased was less than that of CO forest. The content of urea, L-aspartic acid, L-threonine, L-asparagine, L-glutamic acid, γ- aminobutyric acid, and ethanolamine increased in AT forest and that of the other free amino acid decreased or was not detected.
3. Changes in volatile compound content in forestry cultivation sites.
20 volatile compounds were detected in wild garlic planted in five types of forest (Table 5). The most abundant volatile compound was dimethyl trisulfide, followed by disulfide, dimethyl disulfide, methyl 2-propenyl, and 2-hexenal.
The number of volatile compounds detected in wild garlic before planting remained unchanged after it was cultivated in the forests for a year (Table 6). However, in the case of single compounds, there was significant increase or decrease in the content between before and after planting of wild garlic. There was an increase in the content of some volatile compounds after cultivation in the forests. The content of 11, 8, 10, 8, and 4 volatile compounds increased in wild garlic planted in CO, AP, AT, BG, and PK, respectively.
4. Relationship between soil properties and minerals, amino acids, and volatile composition
Soil properties of each experimental site affected minerals, amino acids, and volatile composition (Table 7). pH negatively affected mineral content of wild garlic except manganese. Calcium had positive relationships with total N and cation exchange capacity, whereas sodium, aluminium, iron, and zinc had negative relationships with exchangeable cations.

Pearson’s correlation analysis between soil properties and change in amino acids, minerals and volatile composition.
Amino acid content was strongly affected by pH, exchangeable Ca2+, and Mg2+. In contrast, total N slightly affected amino acid content, which had significant correlations with only 6 of 41 components. Among soil properties, available P and exchangeable Na+ had positive correlation with the content of almost all type of amino acid. However, some amino acid such as L-citrulline, L-α-amino iso-n-butyric acid, cystathionine, L-arginine had no significant correlations with any soil properties.
The volatile compound content was strongly affected by pH, exchangeable Mg2+, whereas available P and cation exchange capacity had little impact on volatile compound compositions: less than only 3 types of volatile compounds had significant correlations with these soil properties. Among volatile compound, disulfide, methyl 2-propenyl, disulfide, methyl 1-propenyl, and tetrasulfide had no significant correlations with any soil properties.
DISCUSSION
1. Effects of forest cultivation on the mineral content of wild garlic
Differences in mineral content were seen between before planting and after planting wild garlic. The most abundant minerals in wild garlic leaves are potassium, calcium, and phosphorus. Among the five regions, the region with the most mineral content was CO forest, followed by PK and AT forest.
It was confirmed that the results of this study were similar to the study by Kim et al. (2012), who reported that among the inorganic components of wild garlic, the potassium and calcium content was very high compared to other inorganic elements. However, the content of inorganic components in wild garlic slightly differed in each research report. Hong and Kim (2018) reported that among the inorganic components of wild garlic, the contents of potassium, calcium, magnesium, phosphorus, sulfur, iron, zinc, and sodium are 24,562.43 ㎎/㎏, 10,538.41 ㎎/㎏, 2,001.38 ㎎/㎏, and 1,656.38 ㎎/㎏, 1,485.71 ㎎/㎏, 203.69 ㎎/㎏, 41.10 ㎎/㎏, and 28.95 ㎎/㎏ respectively, which was said to have a higher content than daylily, slightly different from the content in this study.
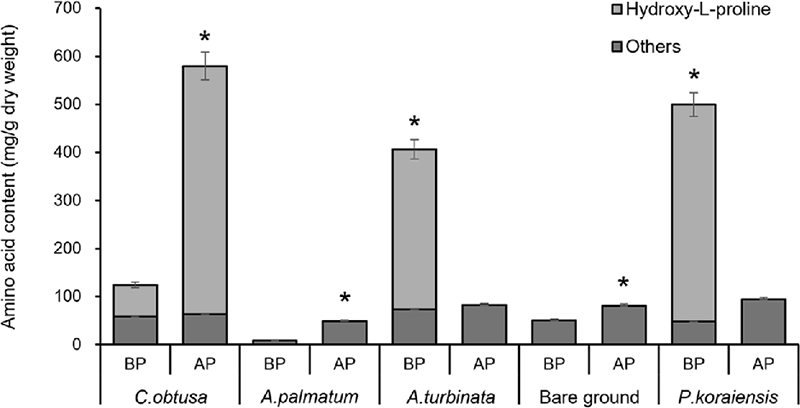
Amino acid content before and after planting wild garlic at five planting sites. Bars indicate means of 3 replications and error bars indicate standard deviations. *Significantly different according to t-test (*p < 0.05).
A significant increase in mineral content was observed in the AT forest, despite its lower levels of available phosphorus and exchangeable cations (K+, Ca2+, Mg2+) compared to other sites. These results suggest that factors beyond soil mineral content, such as total nitrogen content, cation exchange capacity (CEC), and canopy coverage, may have collectively influenced nutrient uptake.
The AT forest exhibited higher CEC and total nitrogen content than other sites, which may have facilitated increased mineral retention and absorption. In nitrogen-rich soils, root growth is enhanced, leading to an expansion of surface area for nutrient uptake, thereby improving mineral absorption efficiency. Additionally, the organic matter content in the AT forest could have played a role in increasing mineral solubility and mobility (Robinson et al., 2005; Oney-Montalvo et al., 2020). Organic acids released during decomposition can enhance the availability of P and other cations.
Canopy structure and shading effects may have also contributed to the increase in mineral content. Wild garlic is sensitive to high temperatures, and shading helps regulate surface temperature, reducing transpiration-induced water stress. Trees with a higher leaf area index (LAI) reduce surface temperature more effectively than those with lower values (Armson et al., 2013). It seems that the trees in AT forest had the largest leaves and LAI among all sites, providing better shading and creating a more favorable microclimate for wild garlic growth.
These findings indicate that soil nitrogen content, CEC, and shading effects may have collectively led to the observed increase in mineral content. However, additional research is necessary to elucidate the precise mechanisms involved. Future studies should examine microbial activity, root structure, and long-term mineral dynamics to better understand these interactions. Additionally, experiments incorporating a broader pH range and controlled fertilizer applications would be beneficial in further investigating soil-plant interactions within forest cultivation systems.
2. Effects of forest cultivation on the free amino acid composition of wild garlic
The amino acid content of wild garlic varied depending on the cultivation environment. The most abundant amino acid in wild garlic was urea, followed by L-asparagine, L-alanine, and L-leucine. This trend was different from the previous studies. Cho et al. (2011) reported that wild garlic's total free amino acid content was 535.39 ㎎/100 g and among the 38 free amino acids isolated, asparagine, glutamic acid, and lysine were contained in high amounts. In addition, Park et al. (1998) reported that the highest free amino acid content in wild garlic were arginine, followed by glutamic acid, and asparagine.
In the CO forest, hydroxy-L-proline content significantly increased, which is a major component of hydroxyproline-rich glycoproteins (HRGPs) involved in cell wall strengthening and stress responses (MacLean et al., 2009; Deepak et al., 2010). This increase could be linked to the allelopathic effects of C. obtusa (Kato-Noguchi et al., 2024) and nitrogen-limited conditions. C. obtusa is known to release secondary metabolites that alter soil chemistry, and the low nitrogen content in the CO forest may have induced stress responses, leading to an increase in hydroxy-L-proline synthesis.
Amino acid synthesis in plants is influenced by soil mineral content. Deficiencies in iron (Fe) and zinc (Zn) have been shown to increase L-asparagine and glutamic acid synthesis, while copper (Cu) and manganese (Mn) deficiencies lead to an overall rise in amino acid levels (Possingham, 1956). Additionally, nitrogen (N) fertilization has been reported to increase arginine, serine, alanine, and γ-aminobutyric acid (Hoff et al., 1974).
In this study, changes in the content of various free amino acids including urea, L-asparagine, L-aspartic acid, γ-aminobutyric acid, and L-glutamic acid were observed, and nitrogen and phosphorus enrichment in AP forest and BG likely promoted amino acid synthesis. In contrast, the D,L-β-aminoisobutyric acid in the CO and PK forest suggests that nitrogen assimilation mechanisms may have been activated under nitrogen-limited conditions.
3. Effects of environmental factors on the volatile compound composition
Change in volatile compound composition in plants occurs in response to various environmental factors, such as soil nutrients (Chrysargyris et al., 2017), foliar diseases (Ponmurugan et al., 2016), and drought stress (Chandrasekaran et al., 2022).
The most abundant volatile compound in wild garlic planted at five sites was dimethyl trisulfide, disulfide, dimethyl, disulfide, methyl 2-propenyl, and 2-hexenal. This result also has some differences from previous studies. The volatile compounds of wild garlic shoot grown in Ulleung Island in Korea are known as dimethyl trisulfide (5.2% - 10.3%), diallyl disulfide (0.1% - 12.1%) and dimethyl pentasulfide (0% - 0.3%), which is somewhat different from this study (Yang et al., 2014). Park et al. (1998) reported that the main volatile compounds in wild garlic from Ulleung Island and Mt. Odae are 3-(methylthio)-I-propene and 2-vinyl-4H-1,3-dithin.
Additionally, in this study, there was no difference in the type and total content of volatile compounds between planting areas, but differences were seen in single compounds. This also is somewhat different from previous studies. Park et al. (1998) found that wild garlic from Mt. Odae or Mt. Jiri contained significantly higher amounts of sulfur compounds than that from Ulleung Island, which has oceanic geographical conditions. In addition, Yang et al. (2014) also reported differences in the types and total contents of volatile compounds between Ulleung Island regions. PCA analysis showed that the habitat of wild garlic plants significantly affected the proportion of alk(en)yl groups (methyl, m-propyl and allyl radicals) in bulb and shoot tissues.
In this study, the contents of amino acids and volatile compounds differed depending on the planting area. Since the study sites were not far apart, the metabolite differences were likely due to differences in upper vegetation. As for upper vegetation, fallen leaves, and compounds released during leaf decomposition enter the soil, affecting wild garlic's growth and metabolite biosynthesis.
Oney-Montalvo et al. (2020) also reported that the physical and chemical composition of the soil affects the profile and concentration of polyphenols, antioxidant activity, and maturity of habanero pepper (Capsicum chinense Jacq.). Additionally, three types of soil significantly affected the concentration of volatile compounds (1-hexanol, hexyl-3-methyl butanoate, 3,3-dimethyl-1-hexanol, and cis-3-hexenyl hexanoate) (Oney-Montalvo et al., 2022).
This study used forestry cultivation method for the efficient production of wild garlic, a useful wild vegetable and medicinal plant. The results indicated that the content of minerals, amino acids, and volatile compounds are affected by the cultivation environment for wild garlic. The mineral content was negatively correlated with pH, which indicates low pH could increase mineral content of wild garlic. High content of available P and exchangeable Na+ in soil led to increase in the content of most of amino acids. The contents of exchangeable cations were related to a decrease in mineral content, whereas amino acids with long retention time were positively correlated with them.
Soil pH influences nutrient availability in soil and nutrient uptake by plants (Barrow and Hartemink, 2023). Normally, low soil pH induces adsorption of anions and cations, reducing nutrient availability. However, uptake of some nutrients, such as phosphorus and sulfur, can increase with decreasing soil pH (Vange et al., 1974; Barrow and Hartemink, 2023). This could have increased the content of most minerals in wild garlic even in low soil pH in this study. However, since the difference in pH among test sites was small (5.0 - 5.4), sites with a wider pH range should be included in forestry cultivation to better determine the effect of soil pH on the mineral content of wild garlic.
Generally, plant can absorb more minerals from soil containing higher content of exchangeable cations. However, the test sites used in this study contained fewer exchangeable cations compared to its native habitat in Ulleung Island, which contained 2.1 ± 1.4 cmolc․㎏-1 Ca2+ and 1.0 ± 0.8 cmolc․㎏-1 Mg2+ (Hur et al., 2012). This led to a decrease in the content of Mg, Ca, Na in leaves after transplanted in almost all test sites, which could distort the results of correlation analysis in this study.
Therefore, soil improvement, such as fertilization and amendment, should be employed to enhance mineral uptake of wild garlic to obtain more accurate and reliable correlation analysis data, which will better reflect the relationship between soil mineral content and plant mineral absorption.
This study found that the content of minerals, amino acids, and volatile compounds differed depending on the environment of forest cultivation. However, this study showed very short-term results, so long-term monitoring is likely necessary. Additionally, analysis of other secondary metabolites (e.g. flavonoid) using liquid chromatography will further support these findings. The results of this study will contribute to a better understanding of the effects of environmental factors on compounds in wild garlic. This will enable the distribution and production of quality wild garlic seeds, as well as the standardization and distribution of other valuable plants in the future.
Acknowledgments
This study was carried out with the support of Korea Forest Service, Mass supply and distribution of promising wild vegetables specialized in the southern region revitalization project(RS-2023-KF002510).
References
-
Armson D, Rahman MA and Ennos AR. (2013). A comparison of the shading effectiveness of five different street tree species in Manchester, UK. Arboriculture and Urban Forestry. 39:157-164.
[https://doi.org/10.48044/jauf.2013.021]
-
Barrow NJ and Hartemink AE. (2023). The effects of pH on nutrient availability depend on both soils and plants. Plant Soil. 487:21-37.
[https://doi.org/10.1007/s11104-023-05960-5]
-
Chandrasekaran, U, Byeon S, Kim K, Kim SH, Park CO, Han AR, Lee YS and Kim HS. (2022). Short-term severe drought influences root volatile biosynthesis in eastern white pine(Pinus strobus L). Frontiers in Plant Science 13:1030140. https://www.frontiersin.org/journals/plant-science/articles/10.3389/fpls.2022.1030140/full, (cited by 2024 June 18)
[https://doi.org/10.3389/fpls.2022.1030140]
- Chang JP, Doh ES, Kil KJ, Yang JK, Yun CW, Lee GH, Jung YH, Ji YS, Kim BR and Choi MS. (2011). Antioxidative activity of A. victorialis var. platyphyllum extracts. Journal of Korean Forest Society. 100:408-416.
-
Cho JY, Park YJ, Oh DM, Rhyu DY, Kim YS, Chon SU, Kang SS and Heo BG. (2011). Amino acid contents and various physiological activities of Allium victorialis. Korean Journal of Plant Resources. 24:150-159.
[https://doi.org/10.7732/kjpr.2011.24.2.150]
- Choi JW, Lee KT, Kim WB, Park KK, Chung WY, Lee JH, Lim SC, Jung HJ and Park HJ. (2005). Effect of Allium victorialis var. platyphyllum leaves on triton WR-1339-induced and poloxamer-407-induced hyper-lipidemic rats and on diet induced obesity rats. Korean Journal of Pharmacognosy. 36: 109-115.
- Choi ST, Lee JT and Park WC. (1993). Growth environment and nutritional evaluation of native Allium victorialis var. platyphyllum in Ulrung island. Applied Biological Chemistry. 36:502-509.
-
Chrysargyris A, Drouza C and Tzortzakis N. (2017). Optimization of potassium fertilization/nutrition for growth, physiological development, essential oil composition and antioxidant activity of Lavandula angustifolia Mill. Journal of Soil Science and Plant Nutrition. 17:291-306.
[https://doi.org/10.4067/S0718-95162017005000023]
-
Cragg GM and Newman DJ. (2013). Natural products: A continuing source of novel drug leads. Biochimica et Biophysica Acta. 1830:3670-3695.
[https://doi.org/10.1016/j.bbagen.2013.02.008]
-
Deepak S, Shailasree S, Kini RK, Muck A, Mithöfer, A and Shetty SH. (2010). Hydroxyproline-rich glycoproteins and plant defence. Journal of Phytopathology. 158:585-593.
[https://doi.org/10.1111/j.1439-0434.2010.01669.x]
- Gold M, Hemmelgarn H, Ormsby-Mori G and Todd C. (2018). Training manual for applied agroforestry practices. University of Missouri Center for Agroforestry. Columbia. MO, USA. p.115-121.
-
Gupta A, Singh PP, Singh P, Singh K, Singh AV, Singh SK and Kumar A. (2019). Medicinal plants under climate change: Impacts on pharmaceutical properties of plants. In Climate change and agricultural ecosystems. Woodhead Publishing. Cambridge, England. p.181-209.
[https://doi.org/10.1016/B978-0-12-816483-9.00008-6]
-
Hoff JE, Wilcox GE and Jones CM. (1974). The effect of nitrate and ammonium nitrogen on the free amino acid composition of tomato plants and tomato fruit. Journal of the American Society for Horticultural Science. 99:27-30.
[https://doi.org/10.21273/JASHS.99.1.27]
-
Hong YS and Kim KS. (2018). Comparative analysis of elemental compositions of selected edible wild plants. Food Science and Preservation. 25:330-336.
[https://doi.org/10.11002/kjfp.2018.25.3.330]
- Hur TC, Yun CW and Joo SH. (2012). Forest site environments and soil properties of Allium victorialis var. platyphyllum in Ullengdo. Journal of Agriculture and Life Science 46:19-26.
-
Imadi SR, Kazi AG, Hashem A, Abd‐Allah EF, Alqarawi AA and Ahmad P. (2015). Medicinal plants under abiotic stress: An overview. In Plant‐environment interaction: Responses and approaches to mitigate stress. John Wiley & Sons. Hoboken, NJ, USA. p.300-310.
[https://doi.org/10.1002/9781119081005.ch16]
- Jeong CH, Ko WH, Cho JR, Ahn CG and Shim KH. (2006). Chemical components of Korean paprika according to cultivars. Food Science and Preservation. 13:43-49.
-
Kato-Noguchi H, Mori K, Iwasaki A and Suenaga K. (2024). Allelopathy and allelochemicals in Chamaecyparis obtusa leaves for the development of sustainable Agriculture. Agronomy 14:1557. https://www.mdpi.com/2073-4395/14/7/1557, (cited by 2025 Feburary 10)
[https://doi.org/10.3390/agronomy14071557]
- Kim GN, Cho MS and Kwon KW. (2010). Analysis growth performance and ascorbic acid contents of Allium victorialis var. platyphyllum, Ligularia fischeri, and L. stenocephala under changing light intensity. Journal of Korean Forest Society. 99:68-74.
-
Kim GN, Han SH, Jang GH and Cho MS. (2015). Physiological responses of the three wild vegetables under different light environment of forest-floor cultivations. Journal of Agriculture and Life Science. 49:19-27.
[https://doi.org/10.14397/jals.2015.49.1.19]
- Kim GT and Um TW. (1997). A study on the distribution of wild edible herb species in Mt. Kariwang. Journal of Korean Forest Society. 86:422-429.
-
Kim JY, Cho JY, Na HS, Choi GC, Park JS, Lee JH, Jeong SH and Moon JH. (2012). Analysis of the various constituents and comparison of biological activities of different parts of Allium victorialis var. platyphyllum. Food Science and Preservation. 44:100-105.
[https://doi.org/10.9721/KJFST.2012.44.1.100]
- Kwon JE, Baek UH, Jung IC and So HY. (2010). Biological activity of fresh juice of wild-garlic, Allium victorialis L. Food Science and Preservation. 17:541-546.
- Lee TB. (1985). Illustrated flora of Korea. Hyangmunsa. Seoul, Korea. p.990.
-
Li Y, Kong D, Fu Y, Sussman MR and Wu H. (2020). The effect of developmental and environmental factors on secondary metabolites in medicinal plants. Plant Physiology and Biochemisty. 148:80-89.
[https://doi.org/10.1016/j.plaphy.2020.01.006]
-
MacLean AM, White CE, Fowler JE and Finan TM. (2009). Identification of a hydroxyproline transport system in the legume endosymbiont Sinorhizobium meliloti. Molecular Plant-Microbe Interactions. 22:1116-1127.
[https://doi.org/10.1094/MPMI-22-9-1116]
- Ministry of Food and Drug Safety(MFDS) (2022). Food code (No. 2022-76, 2022. 10. 25.). Ministry of Food and Drug Safety. Cheongju, Korea. p.392-406.
- National Institute of Agricultural Sciences(NIAS). (2010). Methods of soil chemical analysis. National Institute of Agricultural Sciences. Rural Development Administration. Suwon, Korea. p.1-319.
- National Institute of Forest Science(NIFoS). (2014). Wild garlic cultivation technology. In Forest science news. National Institute of Forest Science. Seoul, Korea. p.14-27.
-
Oney-Montalvo J, Uc-Varguez A, Ramirez-Rivera E, Ramirez-Sucre M and Rodriguez-Buenfil I. (2020). Influence of soil composition on the profile and content of polyphenols in habanero pepper(Capsicum chinense Jacq.). Agronomy. 10:1234. https://www.mdpi.com/2073-4395/10/9/1234, (cited by 2024 June 18)
[https://doi.org/10.3390/agronomy10091234]
-
Oney-Montalvo JE, López-Salas D, Ramirez-Rivera E, Ramirez-Sucre MO and Rodriguez-Buenfil IM. (2020). Influence of soil composition on the profile and content of polyphenols in habanero pepper(Capsicum chinense Jacq.). Horticulturae. 8:428. https://www.mdpi.com/2311-7524/8/5/428, (cited by 2024 June 18)
[https://doi.org/10.3390/agronomy10091234]
- Park HJ, Kim WB, Yoo KO and Jung WT. (1998). Chemical analysis on biologically active substances among habitats of Allium victorialis for a high income crop. Korean Journal of Plant Resources. 11:51-60.
-
Ponmurugan P, Manjukarunambika K and Gnanamangai BM. (2016). Impact of various foliar diseases on the biochemical, volatile and quality constituents of green and black teas. Australasian Plant Pathology. 45:175-185.
[https://doi.org/10.1007/s13313-016-0402-y]
-
Possingham JV. (1956). The effect of mineral nutrition on the content of free amino acids and amides in tomato plants I A comparison of the effects of deficiencies of copper, zinc, manganese, iron, and molybdenum. Australian Journal of Biological Sciences. 9:539-551.
[https://doi.org/10.1071/BI9560539]
-
Robinson B, Bolan N, Mahimairaja S and Clothier B. (2005). Solubility, mobility, and bioaccumulation of trace elements: Abiotic processes in the rhizosphere. In Trace elements in the environment. CRC Press. Boca Raton. FL, USA. p.93-106.
[https://doi.org/10.1201/9781420032048.sec2]
-
Vange MS, Holmern K and Nissen P. (1974). Multiphasic uptake of sulfate by barley roots I. Effects of analogues, phosphate, and pH. Physiologia Plantarum. 31:292-301.
[https://doi.org/10.1111/j.1399-3054.1974.tb03709.x]
-
Verpoorte R and Memelink J. (2002). Engineering secondary metabolite production in plants. Current Opinion in Biotechnology. 13:181-187.
[https://doi.org/10.1016/S0958-1669(02)00308-7]
- Yang JK, Kim JS, Jung JY, Jeong MJ, Song HJ, Yun CW, Kim HH, Do ES, Chang JP, Karigar SC, Choi MS and Moon HS. (2014). Habitat influences composition of volatile constituents in Allium victorialis var. platyphyllum. Pakistan Journal of Botany. 46:271-278.