
고려인삼의 건조 스트레스 처리에 따른 단백질체 비표지 정량분석









This is an open access article distributed under the terms of the Creative Commons Attribution Non-Commercial License (http://creativecommons.org/licenses/by-nc/3.0/) which permits unrestricted non-commercial use, distribution, and reproduction in any medium, provided the original work is properly cited.
Abstract
Korean ginseng (Panax ginseng Meyer) has long been cultivated as an important medicinal plant. Moderate water loss resulting form drought stress can impairs the growth of ginseng and lead to yield reduction. However, the mechanisms by which drought stress affects ginseng at the proteome level remain pooly undersood.
We carried out label-free quantitative proteomic analysis of ginseng roots subjected to drought stress (grown at less than 10% soil moisture for 2 weeks) and, compared the results with a control samples of ginseng grown at 25% soil moisture. The analysis was carried out using liquid chromatography with tandem mass spectrometry. A total of 2,471 proteins were identified, and 195 of which showed significant modulation. Functional classification revealed that proteins involved in calcium signaling, and photosynthesis and, production of secondary metabolites were enriched in the control sample (cluster_1), whereas proteins associated with stress response redox reaction, electron transport, and protein synthesis were enriched in the drought-stressed root (cluster_2).
Our results provide an overview of drought-induced proteomic changes in ginseng root, and illuminate their correlation with physiological changes, revealing potential proteomic markers of drought stress in ginseng.
Keywords:
Abiotic Stress, Drought, Ginseng, Label-free Quantitative Proteomics, LC-MS/MS MaxQuant, Perseus서 언
고려인삼 (Panax ginseng Meyer)은 두릅나무과 (Araliaceae)의 인삼 속에 속하는 다년생 초본식물로서 본래는 재배가 이루어지지 않았던 작물로 최초 재배 시기는 밝혀지지 않았지만, 산에서 자라는 산삼과 구분해서 사용한다 (Ko and Leem, 2009).
한방에서는 일반적으로 뿌리를 인삼이라고 칭하고 있으며, 한국의 대표적 약용작물로 알려져 있다. 건강에 대한 사람들의 관심이 증가함에 따라 생약성분을 다수 포함하고 있는 인삼은 소비가 꾸준히 증가하고 있다. 하지만 최근 지구 평균기온의 상승으로 가뭄 재해가 많이 발생하고 있고, 가뭄은 특히 다른 스트레스들 보다 작물의 생산에 치명적인 영향을 주고 (Cattivelli et al., 2008), 식물의 성장, 발달에 악영향을 주는 등 농업생산량에 큰 장애가 되어 (Boyer, 1982), 인삼 역시 재배에 어려움을 겪고 있다.
인삼은 4 년 - 6 년 동안 재배되기 때문에 타 작물에 비해 스트레스에 더 많이 노출되어 생산이 어렵지만, 특이적인 효능 성분인 산성 다당류와 진세노사이드 (ginsenoside) 등을 갖고 있기에 최적 생육조건과 내부 성분 변화, 스트레스 처리, 유전체 분석 등 여러 분야에서 연구가 꾸준히 진행되고 있다. 인삼은 재배를 통한 생육 과정에서 여러 환경들과 토양의 조건에 따라 재배 수량과 생산물의 상태가 크게 차이가 나는 것으로 보고 되고 있다 (Hyun et al., 2009; Nam et al., 2011; Kang and Kim., 2016).
특히 인삼의 생산량에 영향을 주는 문제 중 하나인 수분 스트레스는 식물체에 루비스코의 활성 억제를 통한 광합성 저하 (Bota et al., 2004), 항산화 효소 변화 (Reddy et al., 2004), 초기 엽록소 사멸 (Mafakheri et al., 2010), 잎 생장저해 (Farooq et al., 2009), H2O2축적 (Neill et al., 2002) 등 여러 생리장해를 끼치는 것으로 알려져 있다 (Lisar et al., 2012). 따라서, 고려인삼의 환경 스트레스에 대한 생리 및 대사를 이해하는 것은 품종 육성과 재배에 중요하다.
인삼의 건조 스트레스에 관한 기존 연구결과들은 수분 스트레스를 처리할 시 생장과 진세노사이드를 비롯한 대사체의 함량 변화에 관한 연구들 (Lee et al., 2005; Eo et al., 2018) 및 인삼의 전사인자 발현 연구와 같은 유전자 수준에 관한 연구 위주로 진행되고 있는 실정이라 (Li et al., 2021), 단백질체 수준의 연구는 부족한 상황이다. 최근 인삼에서 단백질체를 기반으로 진행된 연구는 인삼 부위별 단백질 분석 (Nam et al., 2005) 및 생육 단계별 단백질체 분석 (We et al., 2007; Ma et al., 2013) 등 이 보고된 바 있다.
지난 연구에서는 인삼의 초기 염류 스트레스 처리를 한 뒤 이차원 전기영동 (Two-dimensional gel electrophoresis, 2-DGE) 방법을 활용해 인삼 잎 단백질체 변화 양상을 관찰하였고, 그 결과 초기 염류 스트레스 조건에 의해 감소하는 특이적인 8 개의 단백질을 확인하였다 (Kim et al., 2014).
Van Nguyen 등 (2021)은 최근 인삼의 단백질체 분석 최적화를 위한 인삼 단백질체 추출법의 비교 분석 및 차세대 단백질체 분석의 적용에 대한 결과를 보고한 바 있다. 인삼의 잎, 뿌리, 줄기, 열매 시료를 활용하여 차세대 단백질체 분석을 수행하였고, 총 2,604 개의 통계적으로 유효한 발현 차이를 보이는 단백질을 동정하였다.
하지만 기존의 단백질체 분석은 전통적인 방식인 2-DGE 방법을 통한 연구가 주로 수행되었지만, 2-DGE를 활용한 연구는 분리 동정할 수 있는 단백질 수가 제한적이고 분석에 시간이 많이 소요되어 대량의 단백질체를 정량분석하기 어렵다는 단점이 있다 (Zieske, 2006).
최근에는 고성능 질량분석기 기반의 차세대 단백질체 분석을 활용한 연구가 진행 되고 있고, 2-DGE 방식보다 효율적으로 확인할 수 있도록 해주는 기술이 개발되고 있다 (Van Nguyen et al, 2021). 따라서 고성능 질량분석기 기반의 단백질체 분석은 향후 진행하게 될 인삼연구에서 특정 타겟 단백질들의 발현 및 대사산물 예측의 효율을 높일 수 있는 매우 필수적인 방법이라고 할 수 있다.
따라서 본 연구는 차세대 단백질체 분석법인 비표지 단백질체 정량분석법 (label-free quantitative proteomic analysis)을 이용하여 건조 스트레스 처리를 한 인삼의 뿌리에서 변화하는 단백질을 동정 및 분류하고 기능 분석을 통하여 건조에 대응하는 인삼 단백질체의 변화와 마커 동정을 목적으로 수행하였다 (Fig. 1).
재료 및 방법
1. 실험재료
본 실험에서 사용한 인삼 (Panax ginseng Meyer)은 농촌진흥청 국립원예특작과학원 인삼특작부의 인삼 재배 포장에서 육성 중인 ‘연풍’을 사용하였다. 건조 처리 (drought)는 2년근 인삼을 5 주간 정상 생육시킨 뒤, 토양수분 센서 (WT1000B, RF sensor, Seoul, Korea) 를 사용해 토양수분 10% 이하에서 2 주간 생육하였고, 대조군 (control)은 인삼을 5 주간 정상 생육한 뒤, 토양수분 센서를 사용해 토양수분 25%에서 2 주간 생육 후 뿌리를 수확하여 단백질 분석에 사용하였다. 각 처리구 별 30 개체 이상의 개체를 생리 분석하였고, 단백질제 분석은 3 반복을 수행하였다.
2. Nitro blue tetrazolium (NBT) 및 3,3'-Diaminobenzidine (DAB) 염색
Nitro blue tetrazolium (NBT) 염색 방법은 기존의 연구 방법을 참고해 (Müller et al., 2015) 0.1% NBT를 10 mM potassium phosphate buffer (pH 7.0)에 녹여 2 시간 동안 반응시킨 후 염색하고 실체현미경으로 사진을 찍어 분석하였다. DAB 염색 방법은 기존의 연구 방법 (Kim et al., 2018b)을 참고해 1㎎/㎖ DAB를 10 mM Tris-HCl (pH 7.5)에 녹여 5 시간 반응시킨 후 염색하고 실체현미경 (SZX61 microscope, Olympus Co., Tokyo, Japan)으로 사진을 찍어 분석하였다.
3. 지질 과산화 (lipid peroxidation) 및 hydrogen peroxide (H2O2)측정
지질 과산화 분석은 OxySelect™ TBARS 분석 키트 (STA-330, Cell Biolabs Inc., San Diego, CA, USA)를 이용하여 제조사의 프로토콜을 참고해 수행하였다 (Min et al., 2017).
인삼 분말 0.1 g을 산화를 방지하기 위해 1 × butylated hydroxyltoluene (BHT) solution (최종 농도 0.05%)를 넣은 phosphate-buffered saline (PBS)를 넣어 균질화 한 후 12,000 rpm 및 4℃에서 5 분간 원심분리해 상층액을 얻었다. 얻어낸 상층액에 동일한 부피의 sodium dodecyl sulfate (SDS) lysis buffer를 혼합한 뒤 1 × thiobarbituric acid (TBA) 용액 (각 샘플당 농도 5.2 ㎎/㎖)을 첨가해 샘플을 95℃에서 60 분간 배양했다. 이후 샘플을 실온이 되도록 냉각한 후 1,000 rpm에서 15 분간 원심분리하여 얻은 상층액을 532 ㎚에서 흡광도를 측정한 후, 다음과 같이 malondealdehyde (MDA)의 농도를 계산하였다 (Velikova et al., 2000).
H2O2측정은 Amplex® Red Hydrogen Peroxide/Peroxidase Assay Kit (A2218, Thermo Fisher Scientific Inc., Waltham, MA, USA)를 이용하여 제조업체의 프로토콜 및 지난 연구결과를 참고해 수행하였다 (Min et al., 2017).
H2O2의 활성은 10-acetyl-3,7-dihyfroxyphenoxnazine와 horseradish peroxidase (HRP)을 활용해 측정하였다. 먼저 균질화 한 0.1 g의 시료를 1 × reaction buffer (50 mM sodium phosphate pH 7.4)에 넣어 4℃에서 12,000 rpm으로 5 분간 원심분리한 후 상등액을 얻어낸 뒤 100 μM 10-acetyl-3,7-dihyfroxyphenoxnazine와 0.2 U/㎖ of HRP이 포함된 동일한 부피의 반응용액을 넣어 30 분간 상온에서 배양하였다. 이후 H2O2의 농도를 kit 내에서 제공한 표준곡선을 기반으로 571 ㎚ - 585 ㎚에서 측정한 형광값을 대입해 계산하였다.
4. 단백질 추출
인삼 뿌리 (1 g)를 액체 질소에 넣어 완전히 마쇄한 후, 마쇄된 가루에 2% (v/v) β-mercaptoethanol을 첨가한 Mg/NP-40 단백질 추출용액 [0.5 M Tris-HCl, 2% Nonidet P (NP)-40, 20 mM MgCl2, pH 8.0] 10 ㎖를 첨가하여 균질화 시키고 12,000 rpm으로 4℃에서 10 분간 원심분리를 수행하여 상등액만 획득하였다.
얻어낸 상등액에 4 배 부피의 12.5% (v/v) trichloroacetic acid (TCA)/acetone을 첨가하여 균질화 후 1 일간 -20℃에서 침전시켰다. 침전 진행 후 침전시킨 용액을 4℃에서 12,000 rpm으로 10 분간 원심 분리하여 얻은 침전물을 얻어내고, 그 침전물에 앞서 침전물을 얻은 방법과 동일한 방법으로 2회 다시 원심분리 해 단백질을 세척하였으며 MeOH/chloroform 침전법을 추가적으로 수행하여 단백질 침전물을 세척하였다 (Van Nguyen et al., 2021). 마지막으로 추출된 단백질에 80% acetone 600 ㎕를 첨가하여 micro centrifuge tube에 옮긴 후 -20℃에 보관하였다.
5. 트립신을 이용한 단백질 절편화
정량한 단백질 300 ㎍을 대상으로 filter-aided sample preparation (FASP) 방법을 이용하여 단백질 절편화를 진행하였다 (Gupta et al., 2019; Min et al., 2020).
Acetone에 침전된 단백질 300 ㎍ 을 30 ㎕의 denaturation buffer [4% SDS, 100 mM DTT, 0.1 M tetraethylammonium tetrahydroborate (TEAB), pH 8.5]에 용해시킨 뒤, 3 분간 초음파 분쇄기 처리하고 99℃에서 30 분간 가열한 후, 변성된 단백질을 30K 스핀 필터 (Merck Millipore, Darmstadt, Germany)에 로딩하고 얻어낸 단백질을 최종 300 ㎕의 부피가 되도록 urea buffer (8.0 M urea, 0.1 M TEAB, pH 8.5)를 첨가하였다. 다시 300 ㎕의 urea buffer를 첨가하고 11,180 rpm에서 원심분리를 3 회 반복 진행하여 시료로부터 SDS를 제거하였고, 200 ㎕의 alkylation buffer [50 mM iodoacetamide (IAA), 8.0 M urea, 0.1 M TEAB, pH 8.5]를 첨가하여 상온과 암실조건에서 각각 1 시간동안 시스테인에 알킬화를 진행하였다.
스핀 필터에 TEAB buffer (50 mM TEAB, pH 8.5) 를 넣어 세척한 후 5% acetonitrile에 녹인 트립신 (trypsin gold, mass spectrometry grade, Promega Co., Fitchburg, WI, USA)을 효소와 기질의 비율을 1 : 100 (w/w) 으로 하여 37℃에서 24 시간 동안 처리하여 절편화 하였다.
절편화된 펩타이드들은 원심분리를 통해 얻어내고, 추가적으로 50 mM TEAB와 50 mM NaCl을 이용해 펩타이드를 얻어낸 후, 각 펩타이드의 농도는 Pierce quantitative fluorometric peptide assay kit (Thermo Fisher Scientific Inc., Waltham, MA, USA)를 이용하여 측정하였다.
6. 펩타이드 질량분석 및 통계분석
펩타이드의 염 제거 및 Q-Exactive 장비 (Thermo Fisher Scientific Inc., Waltham, MA, USA)를 활용하여 Liquid Chromatograph-tandem Mass spectrometry (LC-MS/MS) 분석을 수행하였다 (Gupta et al., 2019; Min et al., 2020).
LC-MS/MS분석으로 얻어진 가공 전 데이터는 MaxQuant 소프트웨어 (ver. 1.6.17.0, Max-Planck-Institut für Biochemie, Planegg, Germany)를 사용하여 Panax ginseng database (59,532 entries, http://ginsengdb.snu.ac.kr/)와 비교하였다 (Tyanova et al., 2016a; Jayakodi et al., 2018; Kim et al., 2018a).
Andromeda에서 첫번째에는 20.0 ppm, 다음에는 4.5 ppm의 기본 전구체 질량 허용오차 (default precursor mass tolerances set)를 설정하여 label-free quantification (LFQ) 데이터를 처리했다. LFQ 데이터는 0.5 Da 질량의 허용오차 (최대 2 회 trypsin 절편화를 인식하지 못하는 값)를 기반으로 검색하였다. Cysteine 잔기의 carbamidomethylation 은 고정된 변형을 위해, lysine 잔기의 아세틸화와 methionine 잔기의 산화는 추가적인 변형을 위해 선택되었다. 펩타이드를 식별하기 위해 기존 데이터의 reverse nonsense version을 기반으로 false discovery rate (FDR)를 1%로 설정하였다. 모든 단백질체 질량분석 raw 데이터는 ProteomeXchange Consortium을 통해 PRIDE partner repository에 PXD028602로 등록하였다 (Perez-Riverol et al., 2019).
LFQ 데이터의 통계 분석은 Perseus 소프트웨어 (ver. 1.6.14.0, Max Planck Institute of Biochemistry, Martinsried, Germany)를 사용하여 수행하였다 (Tyanova et al., 2016b). Multiple sample test (One-way ANOVA, Benjamini-Hochberg FDR) 임계값 0.05 이하 및 1.5배 이상의 intensity값 차이를 보이는 단백질체의 선별을 수행하였다 (Kim et al., 2019). 또한 Perseus 소프트웨어 ( ver. 1 .6.14 .0, Max Planck Institute of Biochemistry, Martinsried, Germany)를 활용한 hierarchical clustering analysis (HCA) 및 principle component analysis (PCA)를 추가적으로 수행하였다. 추가적인 단백질체의 기능적 분석을 위하여 AgriGO v2.0 (http://systemsbiology.cau.edu.cn/agriGOv2/) 및 R을 활용한 gene ontology (GO) enrichment 분석, MapMan 분석, CELLO2GO (https://mybiosoftware.com/cello-v-2-5-subcellular-localization-predictor.html)를 활용한 세포 내 단백질체 위치 확인 분석, KEGG brite web-based database를 기반으로 한 KEGG 경로 분석을 각각 수행하였다 (Supek et al., 2011; Tian et al., 2017)
결과 및 고찰
1. 건조 스트레스 처리 인삼의 생리학적 변화 관찰
식물체에 건조 (drought) 처리 시 잎의 생장이 지연되는 현상을 관찰할 수 있으며 (Farooq et al., 2009), 본 연구 결과에서도 동일하게 건조 처리 시 정상 조건의 대조군 (control) 인삼 (Panax ginseng Meyer) 잎에 비하여 잎의 생장이 더 지연되는 것을 관찰하였다 (Fig. 2A).
앞서 관찰한 표현형 결과를 기반으로 NBT와 DAB 염색을 통해 reactive oxygen species (ROS)의 생성 여부를 시각화 하고자 하였다. 염색을 통해 NBT염색 결과로 ROS의 축적을 시각적으로 확인할 수 있었으나 DAB 결과에서는 뚜렷한 차이를 관찰하지 못했다 (Fig. 2A). 뿌리의 지질 과산화 및 ROS의 수치화를 위하여 MDA 및 H2O2의 농도를 측정한 결과, 건조 조건의 인삼 뿌리가 정상 조건의 뿌리에 비해 ROS에 의한 지질 과산화 작용이 유의미하게 증가하고 있음을 확인하였으며 (p < 0.05, Fig. 2B), H2O2 측정에서도 건조 조건의 인삼 뿌리가 대조군 (control)에 비해 H2O2의 측정값이 유의미하게 증가하는 것을 확인하였다 (p < 0.001, Fig. 2C). 이를 통해 인삼 뿌리가 건조 스트레스에 노출되면 세포의 손상을 유발하는 다양한 ROS의 형성과 그를 통한 산화작용이 유도되고 있음을 확인하였다.
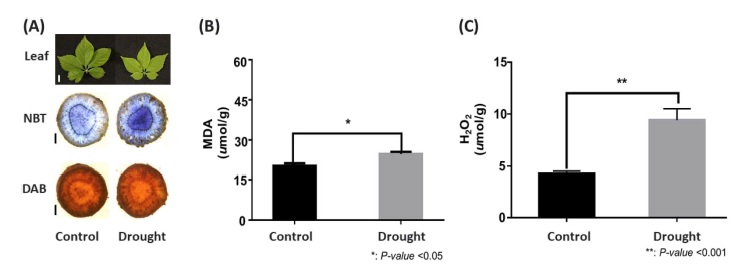
Physiological assessment of drought-induced ROS content in ginseng (var. Yeonpung) root sample.(A); NBT and DAB staining of ginseng plant under control and drought conditions. Bars are 1 ㎝ for leaf, 1 ㎜ for root. (B); Quantitative assessment of malondialdehyde (MDA) under control and drought conditions. (C); Quantitative assessment of H2O2 under control and drought conditions.
식물체의 H2O2 축적은 성장과 발달 및 스트레스와 관련된 다양한 신호전달에 관여하는 주요 인자로 알려져 있다 (Cheeseman, 2007). 일반적으로 염류 스트레스로 인한 ROS의 축적은 광계 2 (photosystem II)의 단백질 함량에 부정적 영향을 주고, 광계 2의 복구를 차단하는 것으로 보고되고 있다 (Messinger et al., 1993; Nishiyama et al., 2001; Allakhverdiev et al., 2002). 인삼의 경우도 비생물적 스트레스 중 하나인 건조 스트레스에 의해 다른 식물체들의 염류 스트레스와 동일하게 식물체 엽록소 내부에 존재하는 광계 2의 복구를 억제시켜, 인삼의 광합성에 영향을 미치는 것으로 생각된다.
2. 건조 스트레스 처리 인삼의 차세대 단백질체 분석
건조 스트레스 처리에 따른 인삼의 단백질체 변화 양상 관찰을 위하여 정상 조건의 인삼과 토양수분 10% 이하로 2 주간 처리한 인삼 뿌리에서 전체 단백질을 추출하였다.
단백질의 절편화 및 LC-MS/MS의 분석을 진행하여 총 2,471 개의 단백질을 동정하였고, 각 반복 내에서 잠재적 오염과 서열이 역으로 인식된 단백질체 및 하나 이상의 펩타이드가 매치되는 2,333 개의 단백질을 최종적으로 확인하였다(Fig. 3A).
대조군 및 건조 처리가 된 두 샘플의 Pearson 상관계수는 모두 0.99 이상의 값으로 각 반복 간의 높은 상관관계를 보이는 것을 확인하였다 (Fig. 3B). 또한 PCA 분석 결과 PC1에서 총 변동의 93.8%로 두 샘플이 구분됨을 확인할 수 있었다(Fig. 3C). 두 샘플 간에 통계적으로 유의미한 발현차이를 보이는 단백질체를 확인하기 위하여, multiple-ANOVA 검정 (Benjamini-Hochberg FDR < 0.05, Fold change > 1.5)을 수행한 결과 총 195 개의 단백질체를 최종적으로 확인하였다 (Fig. 3A).
추가적으로 volcano plot 분석을 수행한 결과 각각 건조 조건에서 증가하는 100 개의 단백질과 건조 조건에서 감소하는 95 개의 단백질로 구분할 수 있었다 (Fig. 3D). 본 분석 방식의 확립은 기존의 인삼 연구에서 부족했던 단백질체 연구를 통한 바이오마커 개발의 기초자료로 제공될 수 있을 것으로 기대된다.
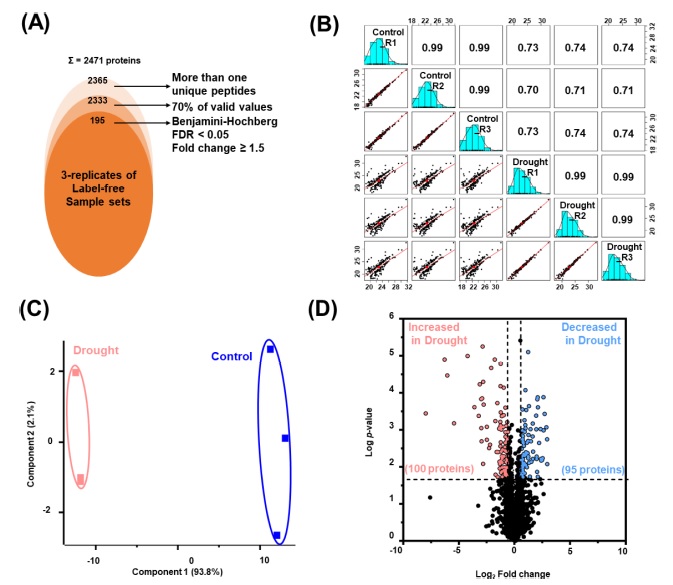
Label-free quantitative proteomic analysis of drought stress treated in ginseng root.(A); venn diagram showing the distribution of the total identified and significantly modulated proteins according to various criteria (identified > 1 peptide, 70% valid values, FDR < 0.05 and Fold change > 1.5). (B); multi-scatterplot showing the expression correlation of log2 transformed LFQ intensity of each sample. Three biological replicates of each sample were used for the statistical test. (C); principle component analysis showing clear separation of 195 significantly modulated proteins. (D); volcano plot highlighting the relative fold change differences of identified proteins from drought and control. Black color indicates significantly modulated proteins during drought stress treatment, the dashed line depicts the fold cutoff value (1.5), blue circles refers to up regulated proteins and red circles correspond to down-regulated proteins.
3. 유의하게 발현되는 단백질체의 기능적 분류 분석
Volcano plot 분석과 마찬가지로 HCA 분석 결과 총 두 개의 cluster로 나뉘는 것을 확인하였다. 각 cluster는 건조 조건에서 감소하는 단백질 (cluster_1)과 건조 조건에 의해 증가하는 단백질 (cluster_2)로 분류되었다 (Fig. 4 A).
유의한 발현 차이를 보이는 단백질들의 역할을 이해하기 위해서, 각 cluster에 해당하는 단백질체의 locus 번호를 활용하여 Uniprot 및 CELLO2GO 웹 기반 데이터베이스를 활용해 단백질 발현위치를 분석하였다. 그 결과, 건조 조건에서 감소하는 95 개의 단백질 중에서는 4 6 개 (48.42%)가 세포질 내에 존재하며, 9 개 (9.47%)가 세포 외부 (extracellular)에 존재하는 단백질이었고, 막 내부와 외부에 존재하는 단백질은 각각 6 개 (6.32%)와 19 개 (20.00%)인 것으로 확인되었다 (Fig. 4B). 또한, 건조 조건에서 증가하는 100 개의 단백질 중 63 개 (63.00%)는 세포질에 위치하는 단백질이었고, 11 개 (11.00%)는 세포 외부에 존재하는 단백질이었다. 그 외에 각각 6 개 (6.00%)와 9 개 (9.00%)의 단백질들은 막 내부와 외부에 존재하는 것으로 확인되었다 (Fig. 4C).
각 cluster에 해당하는 단백질들의 기능 확인을 위하여 GO 분석을 수행하였다. GO 분석 결과 cluster_1에서는 20 종류의 GO term들을 확인할 수 있었으며, 주요 metabolism과 관련된 단백질들 중, 특히 ketone metabolic process와 fruit development와 관련된 단백질들이 건조 조건에서 감소하는 것을 확인 할 수 있었다 (Fig. 4 D).
반면에 건조 처리에 따라 증가하는 단백질 (cluster_2)의 경우 총 41 개의 GO를 확인하였고 비생물적 및 생물적 스트레스 관련된 10 개의 단백질들이 해당 기능과 연관됨을 알 수 있었다. 뿐만 아니라 저 분자 생합성, 질소대사 및 탄수화물대사와 관련된 단백질들이 건조 스트레스 처리에 따라 증가함을 추가적으로 확인할 수 있었다 (Fig. 4E).

Classification of significantly different expressed proteins in drought-treated ginseng root.(A); HCA clustering of significantly modulated proteins by Perseus software. All 195 proteins were grouped by two major clusters based on their abundance patterns among two samples. The result of protein sub-localization analysis of untreated control (B); and drought treated ginseng samples (C); Functional classification of untreated control (D); and drought treated ginseng samples (E); based on cluster expression profiles using GO enrichment database. The size of the circle corresponds to the number of proteins and color scale represents the protein abundance pattern.
기존의 연구에서는 건조 스트레스 처리 시 식물의 과실 발달에 문제가 생겨 최종 과실의 중량과 품질에 영향을 미치는 것으로 보고하였다 (Rahmati et al., 2018). 마찬가지로 해당 연구에서 확인한 단백질체 기능 분석을 통해서도 과실 발달과 영향이 있는 단백질들이 건조 조건에 의하여 감소하는 경향을 보이는 것을 확인할 수 있었다.
수분 관련 스트레스 조건에서는 식물체 내의 광합성이 저하되고 그에 따라 식물 체내의 수용성 탄수화물들의 축적을 촉진시키는 것으로 알려져 있다 (Lee et al., 2002). 해당 연구의 결과는 탄수화물 대사와 관련된 단백질들이 주로 증가하는 경향을 확인하였으며, 이는 앞선 선행 연구의 수분 스트레스 관련 기작에서 보고한 탄수화물 축적 과정과 동일한 메커니즘에 의하여 건조 스트레스 반응이 진행됨을 예측할 수 있었다.
4. 리그닌 대사와 세포벽 분열 관련 단백질의 변화
MapMan 소프트웨어를 활용하여 대조군 및 건조 조건에서 발현 양상이 다른 단백질에 대한 시각화, 기능 분석 및 대사별 분류를 수행한 결과 건조 조건 처리 시 redox, 리그닌 대사, 세포벽의 분열, 광합성, ER-Golgi의 secretary pathway 및 생물적 및 비생물적 스트레스와 관련된 단백질들이 증가 혹은 감소하는 양상을 보이는 것을 확인하였다 (Fig. 5).
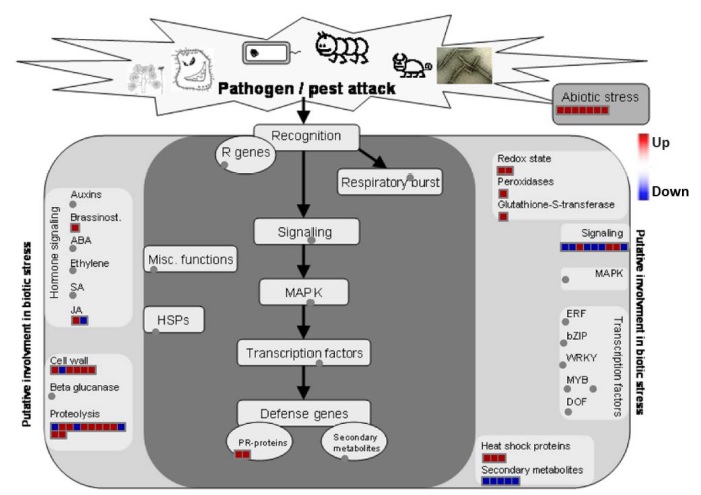
Representative MapMan analysis of significantly expressed proteins in drought stress treatment.Red and blue boxes indicate up-regulated and down-regulated proteins, respectively, in response to drought stress of ginseng.
리그닌은 세포벽 표면에서 합성되는 불균일 중합체이며, 식물 리그닌의 단위체인 모노리그놀은 p-hydroxyphenyl (H), guaiacyl (G), and syringyl (S)로 구성되어 있다 (Besseau et al., 2007). 이전의 연구결과에서는 식물이 건조 스트레스를 받을 시 초기에는 뿌리의 성장을 촉진시키지만 (Yang et al., 2006), 건조 스트레스가 지속될 시 식물의 목본에서 리그닌 생합성을 줄이는 것이 성장에 대한 부정적 영향을 줄이고 식물이 건조 스트레스에 저항하는 방법이라고 보고된 바 있다 (Vincent et al., 2005). 건조 스트레스가 리그닌 합성에 주는 영향은 거의 밝혀져 있지 않지만, 옥수수에서는 건조 스트레스가 리그닌 생합성을 감소시킨다는 것을 과산화효소 활성 감소를 통해 간접적으로 나타낸 적이 있다 (Alvarez et al., 2008).
본 연구 결과에서는 건조 조건의 처리로 인하여 인삼 뿌리 내의 리그닌 대사와 관련된 Phenylalanine ammonia-lyase (Pg_S4658.17)과 RNA binding protein-like protein (Pg_S3838.1) 단백질들이 유의미하게 (1.5 배 이상) 감소하는 것을 확인하였다. Phenylalanine ammonia-lyase (Pg_S4658.17)은 페닐알라닌과 암모니아의 분해에 관여하는 단백질로서 리그닌의 이화과정 뿐만 아니라 생물학적인 방어에도 도움으로 주는 단백질로 보고되고 있으며 (Camm and Towers, 1973), RNA binding protein-like protein (Pg_S3838.1)은 리그닌 생합성과 연관될 뿐만 아니라 세포 내외부의 물 부족과 관련된 생리학적 반응인 기공 운동, abscisic acid (ABA) 자극에 의한 반응 및 세포벽 조직과 관계된 단백질로 알려져 있다 (Lee and Kang, 2016). 따라서 인삼 뿌리의 경우도 마찬가지로 건조 스트레스 처리에 저항하기 위해 리그닌 생합성에 변화를 줄 뿐만 아니라 다양한 세포 내 생리학적 반응을 복합적으로 조절함을 확인할 수 있었다.
세포벽 (cell wall)과 관련된 6 개의 단백질들 중 pectate lyase 1 (Pg_S4713.8)을 제외한 5 개의 단백질들은 건조 처리에 의하여 증가하는 경향을 보이는 것을 확인하였다 (Fig. 5). 특히, 건조 조건에서 감소하는 경향을 보이는 pectate lyase 1은 세포의 막 단백질 중 하나로서 세포벽 발달과 변형에 관련되어 있어 식물의 형태학적 발생을 조절하는 단백질로 알려져 있다 (Uluisik and Seymour, 2020). 특히 식물의 경우 건조 스트레스 조건에서 세포벽의 펙틴 함량을 조절하여 스트레스에 대해 저항하는 것으로 알려져 있다.
5. 광합성 및 secretary pathway 관련 단백질의 변화
건조 조건 인삼 뿌리의 경우, 식물체 내 광합성 경로와 관련된 단백질 Photosystem II CP43 chlorophyll apoprotein (Pg_S2592.5)과 P hotosystem I I D2 p rotein ( Pg_S04 29.2) 등이 감소하는 경향을 보이는 것을 확인하였다.
식물 건조 스트레스 처리 시 탈수로 인하여 용질의 농도가 증가되고 이는 ATP 합성 및 대사 억제를 유발하여 광합성 경로의 진행을 저해 시킨다고 보고된 바 있다 (Kaiser, 1987; Tezara et al., 1999). 또한 식물은 염류, 건조, 열 스트레스를 받았을 시, Ca2+의 유입이 증가하고 ABA 분비량에 변화를 주는 등 손상을 피하기 위해 비슷한 2차 신호전달 과정과 해독과정을 거치는 것으로 알려져 있다 (Xiong and Zhu, 2001; Zhu, 2001; Wahid et al., 2007; Zhang et al., 2012).
본 연구에서는 광합성 경로와 관련된 단백질의 발현 감소뿐 아니라 heat 스트레스 등과 관련된 단백질 heat repeat containing 5B (Pg_S1497.27)은 증가함을 확인할 수 있었다. 또한, endocytosis와 exocytosis와 같은 secretary pathway에 관련된 v-SNARE vesicle-associated membrane protein (VAMP) 7C (Pg_S4374.1)가 확인되었고 스트레스 조건에서 감소되는 경향을 보여주고 있다.
SNARE 단백질의 경우는 고등식물일수록 면역물질의 분비를 수월하게 하기 위해서 더 많은 수의 유전자 수가 존재함이 알려져 있는데 (Sanderfoot, 2007; Kwon et al., 2008), 특히 VAMP의 경우는 식물에서 성장과, flg22 하위 signaling 및 programmed cell death (PCD) 등에 연관되어 식물의 외부스트레스 조건에 대한 저항성과 관련된 단백질로 알려져 있다 (Levine et al., 2001; Yun et al., 2013).
식물의 진균 병원체 감염과 관련하여 v-SNARE 관련 단백질들 혹은 유전자들이 SNARE 매개 endocytosis등을 활용해 진균류에 저항하는 물질을 분비함을 보고한 바 있으며 (Collins et al., 2003), 해당 secretary pathway에 관련된 AtVAMP721/722, OsVAMP714/724 식물의 스트레스 저항성에 있어서 주요한 역할을 수행한다고 보고한 바 있다 (Kwon et al., 2008; Cao et al., 2019). 따라서 인삼의 경우도 해당 경로 관련 단백질인 VAMP 7C (Pg_S4374.1)의 감소하는 경향을 보이고 있으며, 이는 건조 스트레스 조건의 저항성 여부에 직·간접적으로 관여할 것으로 예상된다.
결론적으로 본 연구에서는 인삼 뿌리의 건조 스트레스 조건에 따른 단백질체의 변화 양상의 관찰을 위하여 차세대 단백질체 분석법을 적용하였다. 그 결과 195 개의 유의미한 차이를 보이는 단백질을 동정하고 각 단백질들의 기능을 추가적으로 분석하였다. 기능 분석 결과 건조 스트레스 처리에 의해 통계적으로 유의미하게 증가 혹은 감소하는 단백질들을 확인 할 수 있었으며, 그 중 세포 내의 리그닌 대사, 세포벽 분해, 그리고 광합성 및 세포 내의 ER-Gogi secretary pathway에 관련되는 단백질들을 최종적으로 확인하였다. 본 연구의 결과로 선별해낸 단백질들인 p ectate l yase 1 ( Pg_S4 713.8) 및 VAMP 7C (Pg_S4374.1)와 같은 단백질은 인삼의 건조처리에 따른 세포벽의 변화나 endocytosis 및 면역성의 변화를 단백질 수준에서 확인할 수 있는 마커로 추정되며, 차후 인삼의 건조 스트레스 조건에 대한 단백질 마커로써 적용할 수 있을 것으로 기대된다.
Acknowledgments
본 연구는 농촌진흥청 연구사업(과제번호: PJ01492202)에 의해 이루어진 결과로 이에 감사드립니다.
References
-
Allakhverdiev SI, Nishiyama Y, Miyairi S, Yamamoto H, Inagaki N, Kanesaki Y and Murata N. (2002). Salt stress inhibits the repair of photodamaged photosystem II by suppressing the transcription and translation of psbA genes in synechocystis. Plant Physiology. 130:1443-1453.
[https://doi.org/10.1104/pp.011114]
-
Alvarez S, Marsh EL, Schroeder SG and Schachtman DP. (2008). Metabolomic and proteomic changes in the xylem sap of maize under drought. Plant Cell and Environment. 31:325-340.
[https://doi.org/10.1111/j.1365-3040.2007.01770.x]
-
Besseau S, Hoffmann L, Geoffroy P, Lapierre C, Pollet B and Legrand M. (2007). Flavonoid accumulation in arabidopsis repressed in lignin synthesis affects auxin transport and plant growth. Plant Cell. 19:148-162.
[https://doi.org/10.1105/tpc.106.044495]
-
Bota J, Medrano H and Flexas J. (2004). Is photosynthesis limited by decreased rubisco activity and RuBP content under progressive water stress? New Phytologist. 162:671-681.
[https://doi.org/10.1111/j.1469-8137.2004.01056.x]
-
Boyer JS. (1982). Plant productivity and environment. Science. 218:443-448.
[https://doi.org/10.1126/science.218.4571.443]
-
Camm EL and Towers GHN. (1973). Phenylalanine ammonia lyase. Phytochemistry. 12:961–973.
[https://doi.org/10.1016/0031-9422(73)85001-0]
-
Cao WL, Yu Y, Li MY, Luo J, Wang RS, Tang HJ, Huang J, Wang JF, Zhang HS and Bao YM. (2019). OsSYP121 accumulates at fungal penetration sites and mediates host resistance to rice blast. Plant Physiolgy. 179:1330-1342.
[https://doi.org/10.1104/pp.18.01013]
-
Cattivelli L, Rizza F, Badeck FW, Mazzucotelli E, Mastrangelo AM, Francia E, Mare C, Tondelli A and Stanca AM. (2008). Drought tolerance improvement in crop plants: An integrated view from breeding to genomics. Field Crops Research. 105:1-14.
[https://doi.org/10.1016/j.fcr.2007.07.004]
- Cheeseman J. (2007). Hydrogen peroxide and plant stress: A challenging relationship. Plant Stress. 1:4-15.
-
Collins NC, Thordal-Christensen H, Lipka V, Bau S, Kombrink E, Qiu JL , Huckelhoven R, Stein M , Freialdenhoven A , Somerville SC and Schulze-Lefert P. (2003). SNARE-protein-mediated disease resistance at the plant cell wall. Nature. 425:973-977.
[https://doi.org/10.1038/nature02076]
-
Eo JN, Mo HS and Park KC. (2018). Abiotic factors influencing growth and ginsenoside content of Panax ginseng roots. Horticultural Science and Technology. 36:681-690.
[https://doi.org/10.12972/kjhst.20180068]
-
Farooq M, Wahid A, Kobayashi N, Fujita D and Basra SMA. (2009). Plant drought stress: effects, mechanisms and management. Agronomy for Sustainable Development. 29:185-212.
[https://doi.org/10.1051/agro:2008021]
-
Gupta R, Min CW, Kim YJ and Kim ST. (2019). Identification of msp1-induced signaling components in rice leaves by integrated proteomic and phosphoproteomic analysis. International Journal of Molecular Sciences. 20:1-17. https://www.mdpi.com/1422-0067/20/17/4135, (cited by 2021 Aug 17).
[https://doi.org/10.3390/ijms20174135]
- Hyun DY, Yeon BY, Lee SW, Kang SW, Hyeon GS, Kim YC, Lee KW and Kim SM. (2009). Analysis of occurrence type of physiological disorder to soil chemical components in ginseng cultivated field. Korean Journal of Medicinal Crop Science. 17:439-444.
-
Jayakodi M, Choi BS, Lee SC, Kim NH, Park JY, Jang W, Lakshmanan M, Mohan SVG, Lee DY and Yang TJ. (2018). Ginseng genome database: An open-access platform for genomics of panax ginseng. BMC Plant Biology. 18:62. https://bmcplantbiol.biomedcentral.com/articles/10.1186/s12870-018-1282-9, (cited by 2021 Aug 17).
[https://doi.org/10.1186/s12870-018-1282-9]
-
Kaiser WM. (1987). Effects of water deficit on photosynthetic capacity. Physiologia Plantarum. 71:142-149.
[https://doi.org/10.1111/j.1399-3054.1987.tb04631.x]
-
Kang OJ and Kim JS. (2016). Comparison of ginsenoside contents in different parts of Korean ginseng(Panax ginseng C. A. Meyer). Preventive Nutrition and Food Science. 21:389-392.
[https://doi.org/10.3746/pnf.2016.21.4.389]
-
Kim NH, Jayakodi M, Lee SC, Choi BS, Jang W, Lee J, Kim HH, Waminal NE, Lakshmanan M, van Nguyen B, Lee YS, Park HS, Koo HJ, Park JY, Perumal S, Joh HJ, Lee H, Kim JK, Kim IS, Kim KH, Koduru L, Kang KB, Sung SH, Yu Y, Park DS, Choi D, Seo EY, Kim SG, Kim YC, Hyun DY, Park YI, Kim CS, Lee TH, Kim HU, Soh MS, Lee Y, In JG, Kim H S, K im YM, Yang DC, Wing RA, L ee DY, Paterson AH and Yang TJ. (2018a). Genome and evolution of the shade-requiring medicinal herb Panax ginseng. Plant Biotechnology Journal. 16:1904-1917.
[https://doi.org/10.1111/pbi.12926]
-
Kim SW, Gupta R, Min CW, Lee SH, Cheon YE, Meng QF, Jang JW, Hong CE, Lee JY, Jo IH and Kim ST. (2019). Label-free quantitative proteomic analysis of Panax ginseng leaves upon exposure to heat stress. Journal of Ginseng Research. 43:143-153.
[https://doi.org/10.1016/j.jgr.2018.09.005]
-
Kim SW, Min CW, Gupta R, Jo IH, Bang KH, Kim YC, Kim KH and Kim ST. (2014). Proteomics analysis of early salt-responsive proteins in ginseng(Panax ginseng C. A. Meyer) leaves. Korean Journal of Medicinal Crop Science. 22:398-404.
[https://doi.org/10.7783/KJMCS.2014.22.5.398]
-
Kim YJ, Joo SC, Shi J, Hu C, Quan S, Hu J, Sukweenadhi J, Mohanan P, Yang DC and Zhang D. (2018b). Metabolic dynamics and physiological adaptation of Panax ginseng during development. Plant Cell Reports. 37:393-410.
[https://doi.org/10.1007/s00299-017-2236-7]
- Ko SK and Leem KH. (2009). Discussion of ginseng properties through a historical research of Korean ginseng. The Korea Journal of Herbology. 24:169-172.
-
Kwon C, Neu C, Pajonk S, Yun HS, Lipka U, Humphry M, Bau S, Straus M, Kwaaitaal M, Rampelt H, El Kasmi F, Jürgens G, Parker J, Panstruga R, Lipka V and Schulze-Lefert P. (2008). Co-option of a default secretory pathway for plant immune responses. Nature. 451:835-840.
[https://doi.org/10.1038/nature06545]
- Lee BR, Jung WJ, Kim DH, Kim KY and Kim TH. (2002). Effect of drought stress on carbohydrate composition and concentration in white clover. Korean Journal of Crop Science. 47:48-53.
-
Lee J, Mudge K and Lardner J. (2005). Effect of drought stress on growth and ginsenoside content of american ginseng. HortScience. 16:517-522.
[https://doi.org/10.21273/HORTSCI.40.4.1116A]
-
Lee KU and Kang HS. (2016). Emerging roles of RNA-binding proteins in plant growth, development, and stress responses. Molecules and Cells. 39:179-185.
[https://doi.org/10.14348/molcells.2016.2359]
-
Lee KW, Jung SY, Choi SM and Yang EJ. (2012). Effects of ginsenoside Re on LPS-induced inflammatory mediators in BV2 microglial cells. BMC Complementary Medicine and Therapies. 12:196. https://link.springer.com/article/10.1186/1472-6882-12-196, (cited by 2021 Aug 17).
[https://doi.org/10.1186/1472-6882-12-196]
-
Levine A, Belenghi B, Damari-Weisler H and Granot D. (2001). Vesicle-associated membrane protein of arabidopsis suppresses bax-induced apoptosis in yeast downstream of oxidative burst. Journal of Biological Chemistry. 276:46284-46289.
[https://doi.org/10.1074/jbc.M107375200]
-
Li H, Chen J, Zhao Q, Han Y, Li L, Sun C, Wang K, Wang Y, Zhao M, Chen P, Lei J, Wang Y and Zhang M. (2021). Basic leucine zipper(bZIP) transcription factor genes and their responses to drought stress in ginseng, Panax ginseng C. A. Meyer. BMC Genomics. 22:316. https://bmcgenomics.biomedcentral.com/articles/10.1186/s12864-021-07624-z, (cited by 2021 Aug 17).
[https://doi.org/10.1186/s12864-021-07624-z]
-
Lisar S, Seyed Y, Motafakkerazad R, Mosharraf M, Rahm M and Ismail M. (2012). Water stress plants causes, effect and responses. In water stress. IntechOpen. London, England. p.1-14.
[https://doi.org/10.5772/39363]
-
Ma R, Sun L, Chen X, Jiang R, Sun H and Zhao D. (2013). Proteomic changes in different growth periods of ginseng roots. Plant Physiology and Biochemistry. 67:20-32.
[https://doi.org/10.1016/j.plaphy.2013.02.023]
- Mafakheri A, Siosemardeh A, Bahramnejad B, Struik PC and Sohrabi E. (2010). Effect of drought stress on yield, proline and chlorophyll contents in three chickpea cultivars. Australian Journal of Crop Science. 4:580-585.
-
Messinger J, Schroeder WP and Renger G. (1993). Structure-function relations in photosystem II. Effects of temperature and chaotropic agents on the period four oscillation of flash-induced oxygen evolution. Biochemistry. 32:7658-7668.
[https://doi.org/10.1021/bi00081a009]
-
Min CW, Lee SH, Cheon YE, Han WY, Ko JM, Kang HW, Kim YC, Agrawal GK, Rakwal R, Gupta R and Kim ST. (2017). In-depth proteomic analysis of Glycine max seeds during controlled deterioration treatment reveals a shift in seed metabolism. Journal of Proteomics. 169:125-135.
[https://doi.org/10.1016/j.jprot.2017.06.022]
-
Min CW, Park J, Bae JW, Agrawal GK, Rakwal R, Kim YS, Yang P, Kim ST and Gupta R. (2020). In-depth investigation of low-abundance proteins in matured and filling stages seeds of Glycine max employing a combination of protamine sulfate precipitation and TMT-based quantitative proteomic analysis. Cells. 9:1517. https://www.mdpi.com/2073-4409/9/6/1517, (cited by 2021 Aug 17).
[https://doi.org/10.3390/cells9061517]
-
Muller J, Toev T, Heisters M, Teller J, Moore KL, Hause G, Dinesh DC, Burstenbinder K and Abel S. (2015). Iron-dependent callose deposition adjusts root meristem maintenance to phosphate availability. Developmental Cells. 33:216-230.
[https://doi.org/10.1016/j.devcel.2015.02.007]
-
Nam KY, Park JD and Choi JE. (2011). Radioprotective potential of Panax ginseng: Current status and future prospectives. Korean Journal Medicine Crop Science. 19:287-299.
[https://doi.org/10.7783/KJMCS.2011.19.4.287]
-
Nam MH, Kim SI, Liu JR, Yang DC, Lim YP, Kwon KH, Yoo JS and Park YM. (2005). Proteomic analysis of Korean ginseng(Panax ginseng C. A. Meyer). Journal of Chromatography B. 815:147-155.
[https://doi.org/10.1016/j.jchromb.2004.10.063]
-
Neill S, Desikan R and Hancock J. (2002). Hydrogen peroxide signalling. Current Opinion in Plant Biology. 5:388-395.
[https://doi.org/10.1016/S1369-5266(02)00282-0]
-
Nishiyama Y, Yamamoto H, Allakhverdiev SI, Inaba M, Yokota A and Murata N. (2001). Oxidative stress inhibits the repair of photodamage to the photosynthetic machinery. The EMBO Journal. 20:5587-5594.
[https://doi.org/10.1093/emboj/20.20.5587]
-
Rahmati M, Mirás-Avalos JM, Valsesia P, Lescourret F, Génard M, Davarynejad GH, Bannayan M, Azizi M and Vercambre G. (2018). Disentangling the effects of water stress on carbon acquisition, vegetative growth, and fruit quality of peach trees by means of the qualitree model. Frontiers in Plant Science. 9:3. https://www.frontiersin.org/articles/10.3389/fpls.2018.00003/full, (cited by 2021 Aug 17).
[https://doi.org/10.3389/fpls.2018.00003]
-
Reddy AR, Chaitanya KV and Vivekanandan M. (2004). Drought-induced responses of photosynthesis and antioxidant metabolism in higher plants. Journal of Plant Physiology. 161:1189-1202.
[https://doi.org/10.1016/j.jplph.2004.01.013]
-
Sanderfoot A. (2007). Increases in the number of SNARE genes parallels the rise of multicellularity among the green plants. Plant Physiology. 144:6-17.
[https://doi.org/10.1104/pp.106.092973]
-
Supek F, Bošnjak M, Škunca N and Šmuc T. (2011). REVIGO summarizes and visualizes long lists of gene ontology terms. PLoS One. 6:e21800. https://journals.plos.org/plosone/article?id=10.1371/journal.pone.0021800, (cited by 2021 Aug 17).
[https://doi.org/10.1371/journal.pone.0021800]
-
Tezara W, Mitchell VJ, Driscoll SD and Lawlor DW. (1999). Water stress inhibits plant photosynthesis by decreasing coupling factor and ATP. Nature. 401:914-917.
[https://doi.org/10.1038/44842]
-
Tian T, L iu Y, Yan H, You Q, Yi X, Du Z , Xu W and Su Z . (2017). agriGO v2.0: A GO analysis toolkit for the agricultural community, 2017 update. Nucleic Acids Research. 45:122-129.
[https://doi.org/10.1093/nar/gkx382]
-
Tyanova S, Temu T and Cox J. (2016a). The MaxQuant computational platform for mass spectrometry-based shotgun proteomics. Nature Protocols. 11:2301-2319.
[https://doi.org/10.1038/nprot.2016.136]
-
Tyanova S, Temu T, Sinitcyn P, Carlson A, Hein MY, Geiger T, Mann M and Cox J. (2016b). The Perseus computational platform for comprehensive analysis of (prote)omics data. Nature Methods. 13:713-740.
[https://doi.org/10.1038/nmeth.3901]
-
Uluisik S and Seymour GB. (2020). Pectate lyases: Their role in plants and importance in fruit ripening. Food Chemistry. 309: 125559. https://www.sciencedirect.com/science/article/pii/S0308814619316838, (cited by 2021 Aug 17).
[https://doi.org/10.1016/j.foodchem.2019.125559]
-
Van Nguyen T, Kim SW, Min CW, Gupta R, Lee GH, Jang JW, Divya Rathi D, Shin HW, Jung JY, Jo IH, Hong WJ, Jung KH, Kim S, Kim YJ and Kim ST. (2021). Optimization of protein isolation and label-free quantitative proteomic analysis in four different tissues of Korean ginseng. Plants. 10:1049. https://www.mdpi.com/2223-7747/10/7/1409, (cited by 2021 Aug 17).
[https://doi.org/10.3390/plants10071409]
-
Velikova V, Yordanov I and Edreva A. (2000). Oxidative stress and some antioxidant systems in acid rain-treated bean plants: Protective role of exogenous polyamines. Plant Science. 151: 59-66.
[https://doi.org/10.1016/S0168-9452(99)00197-1]
-
Vincent D, Lapierre C, Pollet B, Cornic G, Negroni L and Zivy M. (2005). Water deficits affect caffeate O-methyltransferase, lignification, and related enzymes in maize leaves. A proteomic investigation. Plant Physiology. 137:949-960.
[https://doi.org/10.1104/pp.104.050815]
-
Wahid A, Gelani S, Ashraf M and Foolad MR. (2007). Heat tolerance in plants: An overview. Environmental and Experimental Botany. 61:199-223.
[https://doi.org/10.1016/j.envexpbot.2007.05.011]
-
We JS, Park HS and Kwon KR. (2007). Proteome analysis of various types of Panax ginseng using 2-dimensional electrophoresis. Journal of Pharmacopuncture. 10:5-18.
[https://doi.org/10.3831/KPI.2007.10.2.005]
-
Xiong L and Zhu J. (2001). Abiotic stress signal transduction in plants: Molecular and genetic perspectives. Physiologia Plantarum. 112:152-166.
[https://doi.org/10.1034/j.1399-3054.2001.1120202.x]
-
Yang L , Wang C, Guo W, L i X, L u M and Yu C . (2006). Differential expression of cell wall related genes in the elongation zone of rice roots under water deficit. Russian Journal of Plant Physiology. 53:390-395.
[https://doi.org/10.1134/S1021443706030150]
-
Yun HS, Kwaaitaal M, Kato N, Yi CH, Park SH, Sato MH, Schulze-Lefert P and Kwon CA. (2013). Requirement of vesicle-associated membrane protein 721 and 722 for sustained growth during immune responses in arabidopsis. Molecular Cells. 35:481-488.
[https://doi.org/10.1007/s10059-013-2130-2]
-
Zhang L, Gao M, Hu J, Zhang X, Wang K and Ashraf M. (2012). Modulation role of abscisic acid(ABA) on growth, water relations and glycinebetaine metabolism in two maize (Zea mays L.) cultivars under drought stress. International Journal of Molecular Science. 13:3189-3202. https://www.mdpi.com/1422-0067/13/3/3189, (cited by 2021 Aug 17).
[https://doi.org/10.3390/ijms13033189]
-
Zhu J. (2001). Plant salt tolerance. Trends in Plant Science. 6:66-71.
[https://doi.org/10.1016/S1360-1385(00)01838-0]
-
Zieske LR. (2006). A perspective on the use of iTRAQTM reagent technology for protein complex and profiling studies. Journal of Experimental Botany. 57:1501-1508.
[https://doi.org/10.1093/jxb/erj168]