
Impact of Dark and Light Treatment on Metabolic Changes and AntioxidantActivities in Adventitious Root Culture of Actinostemma lobatum
This is an open access article distributed under the terms of the Creative Commons Attribution Non-Commercial License (http://creativecommons.org/licenses/by-nc/3.0/) which permits unrestricted non-commercial use, distribution, and reproduction in any medium, provided the original work is properly cited.
Abstract
Tissue culture is an important strategy for metabolite accumulation in plants. Moreover, adventitious roots can produce high levels of phytochemicals. Actinostemma lobatum is consumed as a medicinal plant in South Korea, China, India, and Thailand. In this study, we aimed to identify the effects of darkness and light on metabolic changes and antioxidant activity in the adventitious roots of A. lobatum.
To confirm metabolic changes and antioxidant activity, adventitious roots of A. lobatum were grown under appropriate conditions and separated into dark and light treatment groups. Light conditions were found to more significantly influence the accumulation of natural pigments, such as chlorophyll a [0.136 ± 0.001 ㎎·g-1 dry weight (DW)] and total carotenoids (0.047 ± 0.001 ㎎·g-1 DW), than dark conditions (0.072 ± 0.001 ㎎·g-1 DW and 0.026 ± 0.003 ㎎·g-1 DW, respectively). Moreover, the light treatment group had substantially high contents of total phenolic, flavonoid, and rutin compounds (11.273 ± 0.291 gallic acid equivalent (GAE)·㎎·g-1 DW, 9.943 ± 0.28 quercetin equivalent (QE)·㎎·g-1 DW, and 2.136 ± 0.491 ㎎·g-1 DW, respectively). These results revealed that light stimulates the accumulation of secondary metabolites, such as phenolics and flavonoids, and enhances antioxidant activity in the adventitious roots of A. lobatum.
Light conditions can considerably influence the production of health-beneficial metabolites in the adventitious roots of A. lobatum. This provides an understanding of metabolism in adventitious roots for further experimental studies on environmentally sustainable plant secondary metabolite production. In this study, we show that suitable root cultures have the potential to be used as supplements in the pharmaceutical and nutraceutical industries.
Keywords:
Actinostemma lobatum, Adventitious Root, Exposure of Dark and Light, Phenolics, Antioxidant ActivitiesINTRODUCTION
Actinostemma lobatum is a herbaceous annual plant in Asia, such as South Korea, China, India, and Thailand (Zheng et al., 2020). Historically, the whole plant of A. lobatum was considered a folklore medicinal plant, consumed as a diuretic and for the treatment of diverse chronic diseases (Kim et al., 2008; Cao et al., 2015).
Specifically, several earlier studies reported the anti-oxidative (Kim, 2010), anti-thrombotic (Kim et al., 2008), anti-tumor (Fujioka et al., 1996; Li et al., 2012), antifungal (Choi et al., 2024), and antimicrobial (Chen et al., 2005) activity in A. lobatum extracts, suggesting potential therapeutic applications. Lee et al. (2024) reported that the A. lobatum contained a high level of flavonoids, such as quercetin and kaempferol, which closely correlate with antibiofilm and antihemolytic activities; moreover, Cao et al. (2015) isolated triterpene saponins from A. lobatum, supporting the inhibition activity of the cytotoxicity. Underlying this knowledge, in the present study, we explored the effects of light and dark on adventitious roots of A. lobatum.
In vitro tissue cultures are being developed as a potent strategy for producing biologically useful phytochemicals (Hussain et al., 2022). Especially, the adventitious roots-inducing strategy could enhance the production of pharmaceutically important metabolites (Khanam et al., 2024), following rapid biomass production under sterile conditions (Murthy et al., 2024) without gene modifications.
Additionally, previous studies reported that depending on the incubation conditions, the accumulation of phytochemicals considerably impacted plant roots (Park et al., 2022; Yun et al., 2022). In other words, due to the convenient strategy and high yield of biomass, adventitious root culture, such as eco-friendly and sustainable production of phytochemicals, provided a convenient strategy and high yield of biomass, highlighting the accumulation of secondary metabolites in recent studies.
For the generation and development of plants, environmental factors are essential, including temperature, salt, soil, light, water, pH, and oxygen; moreover, they have significant effects on the production of secondary metabolites (Park et al., 2024). Among them, light is the crucial factor for plant development, controlling the production of plant metabolites (Kapoor et al., 2018).
Several previous studies revealed that light radiation, direction, and intensity cause different accumulations of metabolites in plants (Yang et al., 2018). According to Bungala et al. (2024), different exposed LEDs significantly influenced the biomass, secondary metabolites, and antioxidant activities in Brassica rapa subsp. chinensis, and Park et al. (2024) reported that the light irradiation considerably impacted the production of primary and secondary metabolites in Althaea officinalis hairy root.
In plants, phenolic compounds are a large group of secondary metabolites that are essential in metabolism (Dwivedi et al., 2016) and have been demonstrated to have various activities, such as development, signaling, organogenesis, UV protection, pathogen defense, and response to biotic stress. (Bauters et al., 2021; Pratyusha and Sarada, 2022; Ortiz and Sansinenea, 2023; Kwon et al., 2024).
The biological usage of phenolic compounds, which consist of polyphenols, flavonoids, and phenylpropanoids as antioxidants, is spotlighted, and blocking free radicals and reactive oxygen species (ROS) is thus the subject of numerous studies that have been the subject of efforts at extracting them (Pereira et al., 2016; Porra and Scheer, 2019). In fact, a number of earlier studies have revealed the correlation with phenolic compounds and antioxidant activities (Fu et al., 2011; Lim et al., 2024).
Oxidative stress causes diverse disorder pathogenesis, including cardiovascular diseases, neurological diseases, cancer, and respiratory diseases (Rhee, 2006; Pizzino et al., 2017). As a result, synthetic antioxidants were invented to prevent oxidation in human health, food decay, and the pharmacology industry (Oktay et al., 2003).
However, the side effects of synthetic antioxidants have affected human health; consequently, natural antioxidants have garnered significant interest as alternatives to synthetic antioxidants (Ito et al., 1983; Pokorný, 2007). Hence, numerous studies have revealed the correlation between phytochemicals (phenolics, flavonoids, phenylpropanoids, and carotenoids) and antioxidant activity (Kähkönen et al., 1999; Moure et al., 2001). Based on these considerations, exploration of the natural antioxidants should be conducted for the prevention of disease and treatment associated with oxidative stress.
This study aims to understand the impact of dark and light exposure on compared the antioxidant activity based on differential accumulation of natural pigments and phenolic compounds in adventitious roots of A. lobatum.
In the absence of approaches on the influence of light on the accumulation of phenolics and antioxidant activities in the adventitious roots of A. lobatum, this study could help maximize the accumulation of phytochemicals in tissue cultures and the potential usage as natural antioxidants.
MATERIALS AND METHODS
1. Sample preparation, adventitious root induction, and light treatment
The seeds of the Actinostemma lobatum were collected in October 2023 from Nonsan, Chungcheongnam-do, Korea. A voucher specimen (NNIBRVP122922) was deposited in the Library of Nakdonggang National Institute of Biological Resources (NNIBR, Sangju, Korea). The sterilized seeds of A. lobatum were germinated in a Murashige and Skoog (MS) solid medium (Murashige and Skoog, 1962) at pH 5.8. Seedlings were propagated in a growth chamber using the same medium under 16/8-h light/dark photoperiod at 25℃.
After 4 weeks, a stem segment (0.5 ㎝) was transferred to MS medium with 1.0 ㎎·ℓ-1 indole-3-butyric acid (IBA) and kept in the dark to induce adventitious roots. The root samples from 6-week-old grown A. lobatum were transferred in a Schenk and Hildebrandt (SH) liquid medium (Schenk and Hildebrandt, 1972) with 1.0 ㎎·ℓ-1 IBA and grown on a rotary shaker in the dark for a further 2 weeks.
For light treatment, the acquired adventitious roots were moved into SH liquid medium for 24 h under continuous light at 25℃ from cool white fluorescent; conversely, the control group remained in full darkness for 24 h. After 2 weeks, the samples were harvested, followed by freeze-drying for 72 h. After that, a mortar and pestle were used for grinding the sample, and powder was used for subsequent metabolite analysis and antioxidant activity assays.
2. Assessing chlorophyll and total carotenoid contents from A. lobatum
The chlorophyll and total carotenoid contents (TCC) in adventitious roots of A. lobatum were assessed according to methods from previous reports with slight modifications (Sumanta et al., 2014; Porra and Scheer, 2019). A finely powdered sample was mixed with 99.9% ethanol and incubated at 4℃ for 1 h in the dark. After centrifugation, the supernatant was filtered using PTFE syringe filters, followed by repeating this three times.
Absorbances were read using a UV-vis spectrophotometer (SPECTROstar Nano plate reader, BMG LABTECH., Ortenberg, Baden-Württemberg, Germany), and the contents of chlorophyll and TCC were estimated following equations in Table 1 (Sumanta et al., 2014; Porra and Scheer, 2019).
3. Relative quantification of total phenolic and flavonoid contents
The total phenolic contents (TPC) were estimated by adopting the previous protocol, which monitored the reduction rate of Folin-Ciocalteau reagent, from Lim et al. (2024). The absorbances were measured at 760 nm. The calibration curve of gallic acid (y = 0.0014x – 0.0203, R2 = 0.9997) was used for quantification, and the TPC results were represented in terms of gallic acid equivalent (GAE)·g-1 sample dry weight (DW).
The total flavonoid contents (TFC) were determined, followed by the previously reported method, which is based on flavonoid combining characteristics with aluminum, from Lim et al. (2024). The absorbances were measured at 415 ㎚. The calibration curve of quercetin (y = 0.0017x – 0.0054, R2 = 0.9996) was used for quantification, and the TFC results were presented in terms of quercetin equivalent (QE)·g-1 DW.
4. Determination of individual phenolic compounds by high-performance liquid chromatography (HPLC)
Individual phenolic compounds in adventitious roots of A. lobatum were determined following the protocol described by Lim et al. (2024) with slight modifications. Briefly, 0.1 g of dried sample powder was mixed with 2 ㎖ of 70% MeOH, followed by centrifugation and filtering, and the extracts were directly used for HPLC analysis. The instrument was composed of Agilent 1260 Infinity Ⅱ systems, a C18 column (250 ㎜ × 4.6 ㎜, 5 ㎛, RStech, Daejeon, Korea), and mobile phases of 0.2% acetic acid in distilled water and 99.9% MeOH. The flow rate, column temperature, and wavelength were set to 1 ㎖·min-1, 30℃, and 280 ㎚, respectively. A detailed gradient program was performed following the protocol described by Lim et al. (2024).
5. In vitro antioxidant assays
In vitro antioxidant activities were estimated using 6 different concentrations of sample (ranging from 62.5, 125, 250, 500, 1000, to 2,000 ㎍·㎖-1) and ascorbic acid as a positive control. The 2,2-diphenyl-1-picrylhydrazyl (DPPH) and 2,2'-azino-bis (3-ethylbenzothiazoline-6-sulfonic acid (ABTS) radical scavenging activity in adventitious roots of A. lobatum were performed following a protocol from an earlier report by Lim et al. (2024). The required absolute 50% inhibitory concentration (IC50) was calculated based on dose-response curves plotted using absorbance data and expressed in ㎎·㎖-1. Reducing power was assessed by monitoring the conversion of ferric ion, and methodology was adopted from Lim et al. (2024).
6. Statistical analysis
All values were expressed as the mean ± standard deviation (SD) based on triplicate data. Statistical analysis was performed by using SPSS 20 (SPSS Inc., Chicago, IL, USA), and the significances were determined by the t-test and Duncan’s Multiple Range Test (DMRT) at the 5% level (p < 0.05).
RESULTS
1. Assessment of natural pigment under dark and light exposure
Chlorophyll contents from the adventitious roots of A. lobatum were significantly different under the dark and light-exposed cultures. The adventitious root phenotypic color appeared to be a light brown hue in the dark-grown culture, whereas the light-grown culture showed a greenish color (Fig. 1).
Although there is no significant difference between the dark and light treatment groups in the contents of chlorophyll b, the light-exposed cultures significantly accumulated about 2.0 times higher than dark-exposed cultures in chlorophyll a (p < 0.05) (Table 2). In addition, the light-exposed cultures (0.047 ± 0.001 ㎎·g-1 DW) contained significantly higher TCC than in the dark-exposed cultures (0.026 ± 0.003 ㎎·g-1 DW) (Table 2). From these results, the exposure to dark and light influenced significant changes to natural pigment in the adventitious roots of A. lobatum.
2. Quantification of TPC and TFC contents from A. lobatum
As shown in Table 3, light-exposed adventitious roots had a greater accumulation of TPC and TFC than dark-exposed adventitious roots with significant differences. Specifically, under light conditions, the TPC (11.273 ± 0.291 GAE·㎎·g-1 DW) and TFC (9.943 ± 0.28 QE·㎎·g-1 DW) achieved 1.47 and 2.05 times higher than under the dark conditions (7.665 ± 0.291 GAE·㎎·g-1 DW and 4.855 ± 0.074 QE·㎎·g-1 DW, respectively). These results suggest that light significantly influenced the phenolic and flavonoid contents in the adventitious roots of A. lobatum.
3. Determination of individual phenolic contents
Subsequently, we identified and quantified individual phenolic compounds from the adventitious roots of A. lobatum. Among the identified compounds, (-)-epicatechin, benzoic acid, and kaempferol were not identified as having significant differences; however, sinapic acid that belongs to phenylpropanoid was not detected in the dark-exposed sample, whereas the light-exposed sample was 0.09 ± 0.004 ㎎·g-1 DW. Interestingly, rutin, which belongs to flavonoids, was accumulated higher in light-exposed roots of A. lobatum (2.136 ± 0.491 ㎎·g-1 DW) than in dark-exposed conditions (0.329 ± 0.065 ㎎·g-1 DW).
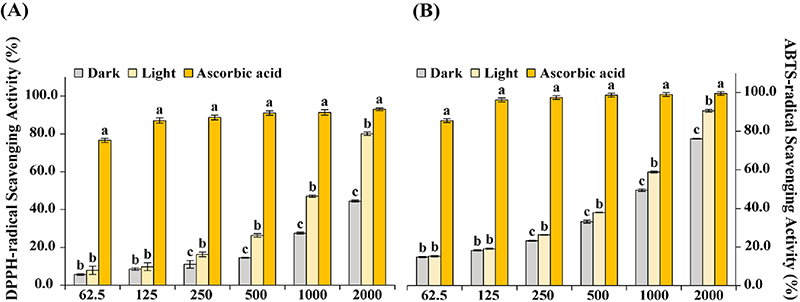
In vitro antioxidant activities from dark and light treated adventitious roots of A. lobatum. Values are the means ± SD, and (A) DPPH-radical scavenging activity, and (B) ABTS-radical scavenging activity. X-axis and Y-axis represent the concentration of extracts (㎍·㎖-1) and inhibition activity (%), respectively. The values with the letter “a” represent the highest using Duncan’s Multiple Range Test (DMRT, p < 0.05).
Although (-)-epicatechin and kaempferol were not confirmed to have a significant difference between dark and light conditions, we can assume that light could induce the accumulation of phenylpropanoid and flavonoids in adventitious roots of A. lobatum.
4. In vitro antioxidant activities
The DPPH, ABTS, and reducing power were efficient assays to evaluate antioxidant activity in a fast and accurate manner and were performed to estimate the effect of dark and light on antioxidant activity from the adventitious roots of A. lobatum.
First, the increase in concentration led to an increase in scavenging activity in both, and the radical scavenging activity of DPPH and ABTS was greater in exposed light samples. Although there were no statistical differences between the dark and light-exposed groups at concentrations of 62.5 and 125 ㎍·㎖-1, statistical differences could be seen at a concentration of 250 ㎍·㎖-1. (Fig. 2).
At a concentration of 2,000 ㎍·㎖-1, in the DPPH assay, light-exposed A. lobatum roots (80.07 ± 0.93%) achieved significantly higher activity than dark-exposed (44.49 ± 0.56%) (Fig. 2A), and similarly in the ABTS assay, exposure to the light (90.56 ± 0.67%) was significantly more influential to scavenging activity than exposure to the dark (76.07 ± 0.29%) (Fig. 2B).
As shown in Table 5, both in DPPH and ABTS, the IC50 value of light-exposed samples (1.157 ± 0.013 ㎎·㎖-1 and 0.88 ± 0.006 ㎎·㎖-1, respectively) was estimated to be higher in efficiency than dark-exposed samples (2.235 ± 0.013 ㎎·㎖-1 and 1.109 ± 0.004 ㎎·㎖-1).
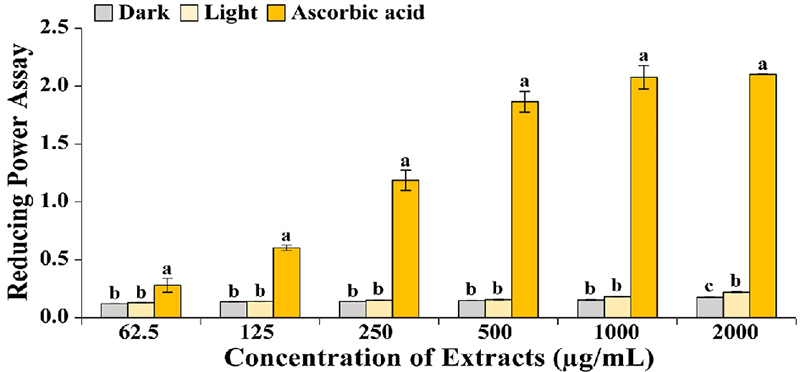
Effects of dark and light on the antioxidant activity of reducing power from adventitious roots of A. lobatum. Results are represented by means ± SD, and the letter “a” shows the highest by One-way ANOVA using Duncan’s Multiple Range Test (DMRT, p < 0.05).
Due to the use of crude extracts, the low sample concentrations likely contained relatively fewer biologically active metabolites. Consequently, no significant differences were observed at lower concentrations. However, a concentration-dependent increase in activity was evident. Notably, at a concentration of 2,000 ㎍·㎖⁻1, light exposure significantly enhanced the reducing power, indicating a higher degree of Fe³⁺ to Fe²⁺ conversion.
Specifically, the adventitious roots of A. lobatum under light conditions (0.22 ± 0.01) showed higher efficiency than under dark conditions (0.18 ± 0.01) (Fig. 3).
Based on these results, the exposure of the light to adventitious roots of A. lobatum significantly influenced the accumulation of phytochemicals, such as chlorophylls, carotenoids, phenolics, flavonoids, and phenylpropanoids, and at the same time, resulted in a higher efficiency of antioxidant activity.
DISCUSSION
Environmental factors significantly influenced not only the generation but also the accumulation of metabolites from plants (Park et al., 2024). In fact, the strategies regulating light have been reported to increase the metabolites in plants (Do et al., 2023; Park et al., 2024). In addition, numerous previous studies have revealed the superiority of inducing adventitious root cultures to produce plant secondary metabolites (Rahmat and Kang, 2019; Khanam et al., 2022).
In this study, we aimed to discover that dark and light caused prominent effects on the accumulation of bioactive compounds, following higher antioxidant activities in A. lobatum adventitious root cultures.
As natural pigments, chlorophylls and carotenoids are well known for various biological activities. In our results, under the light conditions, significantly enhanced chlorophyll and carotenoid contents in adventitious roots of A. lobatum by regulating light-mediated photosynthesis.
Park et al. (2024) demonstrated that the light condition definitely induced the chlorophyll, carotenoid, and phenylpropanoid biosynthetic pathways in hairy roots of A. officinalis, which were consistent with our results. Moreover, Lee et al. (2023) reported that the light treatment significantly impacted the production of rosmaric acid, TPC, antioxidant activities, and antimicrobial activities in Perilla frutescens. Namely, the light treatments are anticipated to induce the production of phytochemicals and beneficial effects in coincidence.
A previous study reported the bioactivity of chlorophyll, such as anti-cancer, interruption of cardiovascular disease, and other chronic diseases (Pareek et al., 2017). Carotenoids defend against oxidative damage to chlorophyll and assist the chlorophyll as photosynthetic pigments (Vechtel et al., 1992; Sumanta et al., 2014). They should be consumed via diet due to their essential features for humans, and numerous studies have revealed the excellent usage of carotenoids as antioxidants (Muller and Bohm, 2011; Jiří et al., 2024).
In other words, exposure to light could lead to the significant accumulation of chlorophyll and carotenoids, resulting in improved photosynthetic systems and their bioactivity.
According to previous reports, they suggested that the light strongly influenced the activation of phenolic biosynthetic pathways (Liu et al., 2021; Yun et al., 2022). Light exposure roots of A. lobatum contained significantly higher secondary metabolites (carotenoids, phenolics, and flavonoids), which are the main scavengers reacting to oxidative stress (Moure et al., 2001).
Carotenoids, phenolics, and flavonoids have had their bioactivity proven by previous reports (Bungala et al., 2024; Jiří et al., 2024; Lim et al., 2024). In detail, sinapic acid and rutin were significantly accumulated under light than dark conditions. Among them, rutin was achieved at approximately 6.49 times higher in light conditions, and Prasad et al. (2019) demonstrated the various bioactive properties (antioxidant, antimicrobial, anti-inflammatory, anticancer, cardioprotective, hepatoprotective, and antidiabetic activity) and the usage in nutraceuticals of rutin.
Light treatment in adventitious roots of A. lobatum considerably contributed to the production of chlorophyll, total carotenoid, phenolic, and flavonoid, and the improved antioxidant activities might be due to the increase of secondary metabolites (Pizzino et al., 2017). Indeed, several previous reports revealed a remarkable correlation between antioxidant activities and total phenolic contents in plants (Kähkönen et al., 1999; Fu et al., 2011).
In conclusion, the light treatment substantially promoted the biosynthesis of phytochemicals and augmented antioxidant responses, indicating its pivotal role in metabolic regulation and antioxidant activity in the adventitious roots of A. lobatum.
This strategy might be helpful for the enhancement of valuable bioactive compounds in the adventitious roots of A. lobatum and utilized for the optimization of in vitro cultures in the adventitious roots of A. lobatum, contributing to the pharmaceutical and nutraceutical industries.
Acknowledgments
This work was supported by a grant from the Nakdonggang National Institute of Biological Resources(NNIBR), funded by the Ministry of Environment(MOE) of the Republic of Korea (NNIBR20253111).
References
-
Bauters L, Stojilković B, Gheysen G. (2021). Pathogens pulling the strings: Effectors manipulating salicylic acid and phenylpropanoid biosynthesis in plants. Molecular Plant Pathology. 22:1436-1448. https://bsppjournals.onlinelibrary.wiley.com/doi/10.1111/mpp.13123, (cited by 2025 Jan. 06).
[https://doi.org/10.1111/mpp.13123]
-
Bungala LTC, Park SU, Nguyen BV, Lim J, Kim K, Kim JK, Park CH, Le AT, Chung YS and Yeo HJ. (2024). Effect of LED lights on secondary metabolites and antioxidant activities in red pakchoi baby leaves. ACS Omega. 9:23420. https://pubs.acs.org/doi/10.1021/acsomega.3c10261, (cited by 2024 Nov. 02).
[https://doi.org/10.1021/acsomega.3c10261]
- Cao J, Wei L, Tang Y, Li N and Zhao Y. (2015). A new cyclic bisdesmoside from Actinostemma lobatum Maxim. Die Pharmazie-An International Journal of Pharmaceutical Sciences. 70:347-350.
- Chen Y, Lin R, Yang S and Gao Z. (2005). Study on the antibacterial activity of Actinostemma tenerum polysaccharides. Preventive Medicine Tribune. 15:1240-1241.
-
Choi S, Lee SH, Hwang BS, Oh YT and Jeon J. (2024). Antifungal activity-guided analysis of Actinostemma lobatum extracts through serial sub-fractions. Plant Pathology Journal. 40:218-224.
[https://doi.org/10.5423/PPJ.NT.11.2023.0152]
-
Do TMH, Choi M, Kim JK, Kim YJ, Park C, Park CH, Park NI, Kim C, Sathasivam R and Park SU. (2023). Impact of light and dark treatment on phenylpropanoid pathway genes, primary and secondary metabolites in Agastache rugosa transgenic hairy root cultures by overexpressing Arabidopsis transcription factor AtMYB12. Life. 13:1042. https://www.mdpi.com/2075-1729/13/4/1042, (cited by 2024 Dec. 28).
[https://doi.org/10.3390/life13041042]
-
Dwivedi SL, Upadhyaya HD, Chung I-M, De Vita P, García-Lara S, Guajardo-Flores D, Gutiérrez-Uribe JA, Serna-Saldívar SO, Rajakumar G, Sahrawat KL, Kumar J and Ortiz R. (2016). Exploiting phenylpropanoid derivatives to enhance the nutraceutical values of cereals and legumes. Frontiers in Plant Science. 7:763. https://www.frontiersin.org/journals/plant-science/articles/10.3389/fpls.2016.00763/full, (cited by 2025 Jan. 17).
[https://doi.org/10.3389/fpls.2016.00763]
-
Fu L, Xu BT, Xu XR, Gan RY, Zhang Y, Xia EQ and Li HB. (2011). Antioxidant capacities and total phenolic contents of 62 fruits. Food Chemistry. 129:345-350.
[https://doi.org/10.1016/j.foodchem.2011.04.079]
-
Fujioka T, Kashiwada Y, Okabe H, Mihashi K and Lee KH. (1996). Antitumor agents 171. Cytotoxicities of lobatosides B, C, D, and E, cyclic bisdesmosides isolated from Actinostemma lobatum maxim. Bioorganic and Medicinal Chemistry Letters. 6:2807-2810.
[https://doi.org/10.1016/S0960-894X(96)00522-7]
-
Hussain MJ, Abbas Y, Nazli N, Fatima S, Drouet S, Hano C and Abbasi BH. (2022). Root cultures, a boon for the production of valuable compounds: A comparative review. Plants. 11:439. https://www.mdpi.com/2223-7747/11/3/439, (cited by 2024 Dec. 27).
[https://doi.org/10.3390/plants11030439]
- Ito N, Fukushima S, Haqiwara A, Shibata M and Ogiso T. (1983). Carcinogenicity of butylated hydroxyanisole in F344 rats. Journal of the National Cancer Institute. 70:343-352.
-
Jiří B, Lenka V, Josef S and Věra K. (2024). Exploring carotenoids: Metabolism, antioxidants, and impacts on human health. Journal of Functional Foods. 118:106284 https://www.sciencedirect.com/science/article/pii/S175646462400286X, (cited by 2024 Dec. 27).
[https://doi.org/10.1016/j.jff.2024.106284]
-
Kähkönen MP, Hopia AI, Vuorela HJ, Rauha J-P, Pihlaja K, Kujala TS and Heinonen M. (1999). Antioxidant activity of plant extracts containing phenolic compounds. Journal of Agricultural and Food Chemistry. 47:3954-3962.
[https://doi.org/10.1021/jf990146l]
-
Kapoor S, Raghuvanshi R, Bhardwaj P, Sood H, Saxena S and Chaurasia OP. (2018). Influence of light quality on growth, secondary metabolites production and antioxidant activity in callus culture of Rhodiola imbricata Edgew. Journal of Photochemistry and Photobiology B: Biology. 183:258-265.
[https://doi.org/10.1016/j.jphotobiol.2018.04.018]
-
Khanam MN, Anis M, Javed SB, Mottaghipisheh J and Csupor D. (2022). Adventitious root culture—an alternative strategy for secondary metabolite production: A review. Agronomy. 12:1178. https://www.mdpi.com/2073-4395/12/5/1178, (cited by 2024 Dec. 27).
[https://doi.org/10.3390/agronomy12051178]
-
Kim KH, Lee HJ, Lee JH, Jang YS, Kim DK, Shim BS, Cho, KH, Ko SG, Ahn KS and Kim SH. (2008). Blockade of glycoprotein IIb/IIIa mediates the antithrombotic activity of butanol fraction of Actinostemma lobatum Maxim. Journal of Ethnopharmacology. 116:431-438.
[https://doi.org/10.1016/j.jep.2007.12.012]
-
Kim DK. (2010). Antioxidative constituents from the whole plant of Actinostemma lobatum Maxim. Journal of the Korean Society for Applied Biological Chemistry. 53:746-751.
[https://doi.org/10.3839/jksabc.2010.113]
-
Kwon H, Sathasivam R, Yoon J, Park C, Park NI, Chung YS and Park SU. (2024). Expression analysis of phenylpropanoid pathway genes and phenylpropanoid accumulation in different organs of Chelidonium majus L. Natural Product Communications. 19:1934578X241239835. https://journals.sagepub.com/doi/full/10.1177/1934578X241239835, (cited by 2024 Dec. 27).
[https://doi.org/10.1177/1934578X241239835]
-
Lee JH, Kim YG, Choi JS, Jeong YT, Hwang BS and Lee J. (2024). Antibiofilm and antihemolytic activities of Actinostemma lobatum extract rich in quercetin against Staphylococcus aureus. Pharmaceutics. 16:1075. https://www.mdpi.com/1999-4923/16/8/1075, (cited by 2025 Jan. 11).
[https://doi.org/10.3390/pharmaceutics16081075]
-
Lee S, Yeo HJ, Lee SY, Kim SR, Park SU and Park CH. (2023). The effect of light and dark treatment on the production of rosmarinic acid and biological activities in Perilla frutescens microgreens. Plants. 12:1613. https://www.mdpi.com/2223-7747/12/8/1613, (cited by 2025 Apr. 02).
[https://doi.org/10.3390/plants12081613]
-
Li W, Cao J, Tang Y, Zhang L, Xie Q, Shen H and Zhao Y. (2012). Cyclic bisdesmosides from Actinostemma lobatum MAXIM(Cucurbitaceae) and their in vitro cytotoxicity. Fitoterapia. 83:147-152.
[https://doi.org/10.1016/j.fitote.2011.10.008]
-
Lim J, Kim K, Kwon DY, Kim JK, Sathasivam R and Park SU. (2024). Effects of different solvents on the extraction of phenolic and flavonoid compounds, and antioxidant activities, in Scutellaria baicalensis hairy roots. Horticulturae. 10:160. https://www.mdpi.com/2311-7524/10/2/160, (cited by 2024 Nov. 19).
[https://doi.org/10.3390/horticulturae10020160]
-
Liu W, Feng Y, Yu S, Fan Z, Li X, Li J and Yin H. (2021). The flavonoid biosynthesis network in plants. International Journal of Molecular Sciences. 22:12824. https://www.mdpi.com/1422-0067/22/23/12824, (cited by 2024 Nov. 19).
[https://doi.org/10.3390/ijms222312824]
-
Moure A, Cruz JM, Franco D, Domı́nguez JM, Sineiro J, Domı́nguez H, Núñez MJ and Parajó JC. (2001). Natural antioxidants from residual sources. Food Chemistry. 72:145-171.
[https://doi.org/10.1016/S0308-8146(00)00223-5]
-
Muller L and Bohm V. (2011). Antioxidant activity of β-carotene compounds in different in vitro assays. Molecules. 16:1055-1069.
[https://doi.org/10.3390/molecules16021055]
-
Murashige T and Skoog F. (1962). A revised medium for rapid growth and bio assays with tobacco tissue cultures. Physiologia Plantarum. 15:473-497.
[https://doi.org/10.1111/j.1399-3054.1962.tb08052.x]
-
Murthy HN, Joseph KS, Paek KY and Park SY. (2024). Bioreactor configurations for adventitious root culture: recent advances toward the commercial production of specialized metabolites. Critical Reviews in Biotechnology. 44:837-859.
[https://doi.org/10.1080/07388551.2023.2233690]
-
Oktay M, Gülçin İ and Küfrevioğlu Öİ. (2003). Determination of in vitro antioxidant activity of fennel(Foeniculum vulgare) seed extracts. LWT-Food Science and Technology. 36:263-271.
[https://doi.org/10.1016/S0023-6438(02)00226-8]
-
Ortiz A and Sansinenea E. (2023). Phenylpropanoid derivatives and their role in plants’ health and as antimicrobials. Current Microbiology. 80:380. https://link.springer.com/article/10.1007/s00284-023-03502-x, (cited by 2024 Nov. 19).
[https://doi.org/10.1007/s00284-023-03502-x]
-
Pareek S, Sagar NA, Sharma S, Kumar V, Agarwal T, González-‐Aguilar GA and Yahia EM. (2017). Chlorophylls: Chemistry and biological functions. In Yahia EM. (ed.). Fruit and vegetable phytochemicals: Chemistry and human health. John Wiley & Sons. Hoboken, NJ, USA. p.269-284.
[https://doi.org/10.1002/9781119158042.ch14]
-
Park YJ, Kim JK, Kim NS, Park YJ, Sathasivam R and Park SU. (2024). Integrated metabolome and transcriptome analyses provide comprehensive insight into dark-and light-responsive mechanisms in Althaea officinalis hairy root cultures. Chemical and Biological Technologies in Agriculture. 11:169. https://link.springer.com/article/10.1186/s40538-024-00698-3, (cited by 2024 Nov. 19).
[https://doi.org/10.1186/s40538-024-00698-3]
-
Park YJ, Kim NS, Sathasivam R, Chung YS and Park SU. (2022). Impact of copper treatment on phenylpropanoid biosynthesis in adventitious root culture of Althaea officinalis L. Preparative Biochemistry & Biotechnology. 52:283-291.
[https://doi.org/10.1080/10826068.2021.1934697]
-
Pereira C, Barros L and Ferreira IC. (2016). Extraction, identification, fractionation and isolation of phenolic compounds in plants with hepatoprotective effects. Journal of the Science of Food and Agriculture. 96:1068-1084.
[https://doi.org/10.1002/jsfa.7446]
-
Pizzino G, Irrera N, Cucinotta M, Pallio G, Mannino F, Arcoraci V, Squadrito F, Altavilla D and Bitto A. (2017). Oxidative stress: harms and benefits for human health. Oxidative Medicine and Cellular Longevity. 2017:8416763. https://onlinelibrary.wiley.com/doi/10.1155/2017/8416763, (cited by 2024 Dec. 15).
[https://doi.org/10.1155/2017/8416763]
-
Pokorný J. (2007). Are natural antioxidants better–and safer–than synthetic antioxidants? European Journal of Lipid Science and Technology. 109:629-642.
[https://doi.org/10.1002/ejlt.200700064]
-
Porra RJ and Scheer H. (2019). Towards a more accurate future for chlorophyll a and b determinations: the inaccuracies of Daniel Arnon’s assay. Photosynthesis Research. 140:215-219.
[https://doi.org/10.1007/s11120-018-0579-8]
-
Prasad R and Prasad SB. (2019). A review on the chemistry and biological properties of rutin, a promising nutraceutical agent. Asian Journal of Pharmacy and Pharmacololgy. 5:1-20.
[https://doi.org/10.31024/ajpp.2019.5.s1.1]
-
Pratyusha DS and Sarada DVL. (2022). MYB transcription factors—master regulators of phenylpropanoid biosynthesis and diverse developmental and stress responses. Plant Cell Reports. 41:2245-2260.
[https://doi.org/10.1007/s00299-022-02927-1]
-
Rahmat E and Kang Y. (2019). Adventitious root culture for secondary metabolite production in medicinal plants: A review. Journal of Plant Biotechnology. 46:143-157.
[https://doi.org/10.5010/JPB.2019.46.3.143]
-
Rhee SG. (2006). H2O2, a necessary evil for cell signaling. Science. 312:1882-1883.
[https://doi.org/10.1126/science.1130481]
-
Schenk RU and Hildebrandt A. (1972). Medium and techniques for induction and growth of monocotyledonous and dicotyledonous plant cell cultures. Canadian Journal of Botany. 50:199-204.
[https://doi.org/10.1139/b72-026]
- Sumanta N, Haque CI, Nishika J and Suprakash R. (2014). Spectrophotometric analysis of chlorophylls and carotenoids from commonly grown fern species by using various extracting solvents. Research Journal of Chemical Science. 4:63-69.
- Vechtel B, Eichenberger W and Ruppel G. (1992). Lipid bodies in Eremosphaera viridis De Bary(Chlorophyceae). Plant and Cell Physiology. 33:41-48.
-
Yang L, Wen KS, Ruan X, Zhao YX, Wei F and Wang Q. (2018). Response of plant secondary metabolites to environmental factors. Molecules. 23:762. https://www.mdpi.com/1420-3049/23/4/762, (cited by 2025 Jan. 13)
[https://doi.org/10.3390/molecules23040762]
-
Yun C, Zhao Z, Gu L, Zhang Z, Wang S, Shi Y, Miao N, Ri I, Wang W and Wang H. (2022). In vitro production of atractylon and β-eudesmol from Atractylodes chinensis by adventitious root culture. Applied Microbiology and Biotechnology. 106:7027-7037.
[https://doi.org/10.1007/s00253-022-12194-5]
-
Zheng L, Zhang T, Xie L, Karrar E, Shi L, Jin J, Wang X and Jin Q. (2020). Physicochemical characteristics of Actinostemma lobatum Maxim. kernel oil by supercritical fluid extraction and conventional methods. Industrial Crops and Products. 152:112516. https://www.sciencedirect.com/science/article/abs/pii/S0926669020304325, (cited by 2024 Dec. 15).
[https://doi.org/10.1016/j.indcrop.2020.112516]