
Isolation of Guaian-6,12-olides from Artemisia princeps and Their Antioxidant Activity
This is an open access article distributed under the terms of the Creative Commons Attribution Non-Commercial License (http://creativecommons.org/licenses/by-nc/3.0/) which permits unrestricted non-commercial use, distribution, and reproduction in any medium, provided the original work is properly cited.
Abstract
Korean mugwort, Artemisia princeps, a plant that has been widely used in diet and traditional medicine throughout East Asia.
Two novel guaian-6,12-olides (1 and 2) and two known compounds (3 and 4) were isolated from Korean mugwort, their chemical structures were established by extensive spectroscopic analysis and comparison with those of known compounds. The antioxidant capacity of the isolated compounds was evaluated by measuring intracellular reactive oxygen species (ROS) generation in HT-1080 cells and the extent of oxidative damage to genomic DNA purified from HT-1080 cells. Compounds 1 and 3 exhibited outstanding intracellular ROS scavenging activity at a concentration of 10 μM compared to the untreated blank. Particularly, 3 exhibited an exceptional ROS scavenging activity of approximately 67% at a concentration of 1 μM after 2 h. Additionally, all compounds significantly inhibited DNA oxidation induced by hydroxyl radicals at a concentration greater than 1 μM. Among them, 4 showed the most effective protection against DNA damage.
Further studies are expected to provide more insight on development of guaian-6,12-olides as potential antioxidants and mechanism by which these types of guaianolides act as antioxidants.
Keywords:
Artemisia princeps, DNA oxidation, Guaian-6,12-olides, Intracellular ROSINTRODUCTION
To date, over 10,000 isolated sesquiterpene lactones have been identified in numerous plant species, including members of Solanaceae, Euphorbiaceae, Lauraceae, and Magnoliaceae families (Karadeniz et al., 2021).
However, most sesquiterpene lactones, which are known for their bioactivity, have been isolated from the Asteraceae family. These compounds exhibit diverse structural features but share a common γ-lactone ring, and they are primarily classified into five major groups based on their carbocyclic skeleton: eudesmanolides, germachronolides, guaianolides, pseudoguaianolides, and xanthanolides (Moujir et al., 2020).
Guaianolide is a sesquiterpene lactone characterized by the presence of a gamma lactone and either a cyclopentane or cyclopentene, which unite to form a central cycloheptane or cycloheptene moiety (Dhyani et al., 2022).
Based on the attachment point of the lactone to the central ring, two types of olides are known: guaian-6,12-olides and guaian-8,12-olides. Some guaianolides exhibit a variety of biological activities, including antitumor, antiviral, antibacterial, antifungal, hepatoprotective, anti-inflammatory, antiulcer, anthelmintic, contraceptive, and sedative effects (Vajs et al., 1999; Wedge et al., 2000; Drew et al., 2009; Ma et al., 2019; Salazar-Gómez et al., 2020; Nawrot et al. 2021; Dhyani et al., 2022).
Artemisia princeps Pampanini, a vascular plant of the Asteraceae family that is covered by spider web-like hairs, grows perennially around sunny farmlands and forest edges. This plant has been widely used for medicinal purposes in East Asian regions such as Korea, Japan, and Manchuria in China, and the new leaves that grow in spring are also used for food (Bang et al., 2007; Li et al., 2011).
During a search for bioactive compounds from natural resources, two new guian-6,12-olides, artemprilactones A and B (1 and 2), along with two known compounds (3 and 4), were isolated from Artemisia princeps. In this study, we report the isolation, structural determination, and antioxidant activity of these compounds.
MATERIALS AND METHODS
1. Apparatus and reagents
1H and 13CNMR spectra were recorded using a Bruker Avance II NMR 900 spectrometer (Billerica, MA, USA). 1H and 13C spectra were measured using standard Bruker pulse sequence programs at 900 and 225 MHz, respectively. All chemical shifts were recorded for the residual methanol-d4 (Cambridge Isotope Laboratories, Inc., Cambridge, MA, USA). high-resolution electrospray ionisation mass spectrometry (HR ESI-MS) analysis was performed at the Korea Basic Science Institute (Ochang, Korea) Center of Research Equipment.
High-performance liquid chromatography (HPLC) was performed using a Dionex P580 system (Dionex corporation, Sunnyvale, CA, USA) equipped with a Varian 350 RI detector.
The setup included a YMC pack ODS-A column (250 ㎜ × 10 ㎜, Tokyo, Japan) and an Alltech guard column (7.5 ㎜ × 4.6 ㎜, HICHROM Theale, England). All solvents utilized were either of spectral-grade quality or distilled from glass before their application.
2. Isolation of guian-6,12-olides (1–4) from A. princeps
Samples of A. princeps collected from the seashore of Donggeom Island, Ganghwagun, Incheon, in 2012 were dried in the shade and immediately cut into small pieces for extraction with organic solvents.
The extraction solvents used were relatively nonpolar CH2Cl2 and polar MeOH to maximize the extraction of both nonpolar and polar components. First, the samples were immersed in CH2Cl2 and extracted at room temperature for two days.
After filtering the extraction solution, the residue was subjected to the same process again using MeOH solvent. The obtained extraction solutions were concentrated individually, and the resulting residues were combined to yield a total crude extract of 146.2 g. The combined crude extracts were subjected to initial fractionation using CH2Cl2 and water.
The separated organic layer was fractionated using n-hexane and 85% aqueous MeOH (85% aq.MeOH), producing n-hexane and 85% aq.MeOH fractions of 14.3 g and 28.9 g, respectively. The aqueous layer was also re-fractionated into n-BuOH and water, yielding n-BuOH and H2O fractions of 42.6 g and 60.8 g.
A portion of the 85% aq. MeOH fraction was divided into seven subfractions (rf1~7) using a gradient mixture of methanol and water (50%, 60%, 70%, 80%, and 90% aq. MeOH, 100% MeOH) and finally 100% EtOAc as the eluent for C18 reversed-phase column chromatography. Among these fractions, the 60% aq. MeOH sub-fraction was further subjected to reversed-phase HPLC (using ODS-A and 45% aq. MeOH) to obtain the four guaian-6,12-olides.
Artemprilactone A (1): [α]D20 +5.62 (c 0.65, MeOH); 1H NMR (900 MHz, CD3OD) δ 5.50 (1H, s, H-3), 5.22 (1H, s, H-14), 5.11 (1H, s, H-14), 4.85 (1H, dt, J=4.8, 10.4 Hz, H-8), 3.98 (1H, t, J=10.4 Hz, H-6), 2.84 (1H, d, J=16.2 Hz, H-2), 2.68 (1H, dd, J=12.0, 10.4 Hz, H-9), 2.66 (1H, d, J=10.4 Hz, H-5), 2.58 (1H, m, H-11), 2.53 (1H, dd, J=12.0, 4.8 Hz, H-9), 2.46 (1H, q, J=10.4 Hz, H-7), 2.39 (1H, d, J=16.2 Hz, H-2), 2.08 (3H, s, CH3COO–), 1.81 (3H, s, H-15), 1.25 (3H, d, J=7.1 Hz, H-13); 13C NMR (75 MHz, CD3OD) δ 180.3 (C-12), 171.6 (C, –OAc), 147.0 (C-10), 140.6 (C-4), 125.7 (C-3), 117.0 (C-14), 85.0 (C-1), 80.9 (C-6), 77.9 (C-8), 64.9 (C-5), 54.2 (C-7), 46.3 (C-2), 41.8 (C-11), 37.9 (C-9), 21.2 (CH3CO-), 17.7 (C-15), 15.8 (C-13); HMBC correlations H-2/C-1, C-3, C-4; H-3/C-1, C-2, C-5; H-5/C-1, C-3, C-4, C-6; H-6/C-4, C-8; H-7/C-5, -6, -8, -9, -11, -13; H-8/C-11, -CO-Me; H-9/C-1, C-7, C-8, C-10, C-14; H-11/C-7, -8, -12, -13; H-13/C-7, C-11, C-12; H-14/C-1, C-9, C-10; H-15/C-3, C-4, C-5; HR ESI-MS m/z: 329.1366[M + Na]+(calcd for C17H22O5Na 329.1365).
Artemprilactone B (2): [α]D20 +13.24 (c 0.65, MeOH); 1H NMR (900 MHz, CD3OD) δ 5.46 (1H, s, H-3), 5.42 (1H, dt, J=10.5, 2.0 Hz, H-8), 5.31 (1H, dt, J=2.0, 1.5 Hz, H-9), 4.06 (1H, dd, J=10.5, 10.5 Hz, H-6), 2.62 (1H, m, H-11), 2.61 (1H, d, J=10.5 Hz, H-5), 2.54 (2H, m, H-2), 2.45 (1H, q, J=10.5 Hz, H-7), 2.08 (3H, s, COOCH3), 1.89 (6H, brs, H-14/-15), 1.26 (3H, d, J=7.0 Hz, H-12); 13C NMR (75 MHz, CD3OD) δ 180.2 (C-13), 170.6 s (C, COOCH3), 143.2 (C-4), 141.5 (C-10), 124.2 (C-3), 123.6 (C-9), 83.6 (C-1), 80.9 (C-6), 75.3 (C-8), 66.3 (C-5), 52.9 (C-7), 47.1 (C-2), 42.0 (C-11), 24.4 (C-14), 21.1 (CH3CO–), 18.3 (C-15), 15.9 (C-12); HR ESI-MS m/z: 329.1368[M + Na]+(calcd for C17H22O5Na 329.1365).
Neoezoguaianin (3): [α] D20 +1.47 (c 0.68, MeOH); 1H NMR (900 MHz, CD3OD) δ 5.88 (1H, d, J=5.7 Hz, H-3), 5.54 (1H, d, J=5.7 Hz, H-2), 5.09 (1H, s, H-14), 4.87 (1H, s, H-14), 4.88 (1H, m, H-8), 4.26 (1H, dd, J=11.0, 9.3 Hz, H-6), 2.87 (1H, dd, J=11.1, 11.1 Hz, H-9), 2.71 (1H, q, J=10.1 Hz, H-7), 2.60 (2H, m, H-10/-11), 2.33 (1H, d, J=11.0 Hz, H-5), 2.09 (3H, s, COOCH3), 1.30 (3H, d, J=7.1 Hz, H-12), 1.27 (3H, s, H-15); 13C NMR (75 MHz, CD3OD) δ 180.4 (C-13), 171.6 (C, COOCH3), 146.2 (C-10), 141.1 (C-3), 135.4 (C-2), 116.9 (C-14), 85.9 (C-1), 83.0 (C-4), 79.5 (C-6), 77.9 (C-8), 66.9 (C-5), 51.8 (C-7), 41.4 (C-11), 41.4 (C-11), 37.5 (C-9), 24.1 (C-15), 21.1 (CH3, COOCH3), 16.6 (C-12); HR ESI-MS m/z: 345.1315[M + Na]+(calcd for C17H22O6Na 345.1314).
Ezoartemin (4): [α]D20 +1.32 (c 0.71, MeOH); 1H NMR (900 MHz, CD3OD) δ 5.11 (1H, ddd, J=10.1, 7.2, 7.2 Hz, H-8), 4.18 (1H, dd, J=10.5, 10.5 Hz, H-6), 3.70 (1H, d, J=1.1 Hz, H-2), 3.36 (1H, d, J=1.1 Hz, H-3), 2.82 (1H, q, J=11.1 Hz, H-7), 2.81 (1H, d, J=11.0 Hz, H-5), 2.61 (1H, m, H-11), 2.21 (1H, dd, J=14.9, 7.0 Hz, H-9), 2.05 (3H, s, COOCH3), 1.68 (1H, dd, J=14.9, 7.4 Hz, H-9), 1.47 (3H, s, H-15), 1.30 (3H, d, J=6.9 Hz, H-12), 1.03 (3H, s, H-14); 13C NMR (75 MHz, CD3OD) δ 180.2 (C-13), 171.4 (C, COOCH3), 79.9 (C-6), 78.6 (C-1), 72.6 (C-8), 72.4 (C-4), 70.3 (C-10), 58.9 (CH, C-3), 58.2 (C-2), 53.0 (C-7), 47.2 (C-9), 44.1 (C-5), 41.5 (C-11), 27.0 (C-14), 21.0 (CH3, COOCH3), 20.0 (C-15), 15.9 (C-12); HR ESI-MS m/z: 361.1263[M + Na]+(calcd for C17H22O7Na 361.1263).
3. Cell culture
The fibrosarcoma HT-1080 cells (Korean Cell Line Bank) employed in the experiment were cultured at 37°C in a 5% CO2 incubator (Thermo Scientific™Forma™ , Waltham, MA, USA) using RPMI-1640 and Dulbecco's Modified Eagle's Medium (DMEM) (Hyclone, Logan, UT, USA) supplemented with 100 units/㎖ penicillin–streptomycin (GIBCO, Waltham, MA, USA).
The following steps were carried out 2–3 times per week: the cultured cells were washed with 1 × phosphate buffered saline (PBS), and the medium was replaced. The attached cells were detached using 0.05% trypsin–0.02% ethylenediaminetetraacetic acid (EDTA) solution and subsequently sub-cultured for further propagation.
4. Cell viability measurement
Cell viability was evaluated using a modified Mosmann's method (Mosmann, 1983).
HT-1080 cells were seeded at a density of 1 × 104 cells/well in a 96-well plate, treated with various sample concentrations and incubated for 24 hours. The cultured cells were then washed with fresh medium and exposed to a solution containing 3-(4,5-dimethylthiazol-2-yl)-2,5-diphenyl tetrazolium bromide (MTT) at a concentration of 1 ㎎/㎖ MTT for 4 hours. After the culture medium was removed, the generated formazan was solubilized using dimethyl sulfoxide (DMSO), and the absorbance was measured at 540 ㎚.
5. Determination of the intracellular formation of ROS using DCF-DA labeling
Intracellular production of ROS was evaluated using the oxidation-sensitive dye 2’,7’-dichlorofluorescein diacetate (DCFH-DA) as the substrate.
HT-1080 cells cultured in fluorescence microtiter 96-well plates were exposed to 20 μM DCFH-DA in HBSS and allowed to incubate for 20 min in darkness. DCFH-DA, a non-fluorescent dye capable of freely entering cells, undergoes hydrolysis by intracellular esterases to form DCFH, which then accumulates within the cells. Subsequently, the cells were treated with various test samples and incubated for 1 h. Following three washes with PBS, the cells were exposed to 500 μM H2O2 dissolved in Hanks' balanced salt solution (HBSS).
The production of DCF, a fluorescent compound (λexcitation = 485 ㎚; λemission = 528 ㎚), resulting from the oxidation of DCFH in the presence of various ROS, was monitored at 30 min intervals utilizing a multidetection microplate fluorescence spectrophotometer (Synergy HT, Bio-Tek Instruments, Winooski, VT, USA).
The temporal effects of the sample groups were plotted and compared with the fluorescence intensity of the control and blank groups (Okimotoa et al., 2000).
6. Genomic DNA isolation and determination of radical mediated DNA damage
The genomic DNA used in the experiment was extracted from HT-1080 cells using the AccuPrep® Genomic DNA Extraction kit (Bioneer Inc., Oakland, CA, USA).
The purity and concentration of the extracted genomic DNA were quantified by measuring its absorbance at wavelengths of 260 and 280 ㎚, and then stored at –20℃ for later use.
To oxidize the genomic DNA, 4 ㎕ of a compound, along with 600 μM of FeSO4 and 0.5 mM of H2O2, were added to 0.5 ㎍ - 1.0 ㎍ of genomic DNA, and the mixture was allowed to oxidize at room temperature for 30 min. The reaction was stopped by adding 130 mM EDTA. Oxidized genomic DNA was loaded onto a 1% agarose gel containing 6 × agarose gel loading buffer and subjected to electrophoresis at 100 V for 30 min. After electrophoresis, the gel was stained with 1 ㎎/㎖ ethidium bromide solution and visualized under UV light using a CAS-400SM Davinch-ChemiimagerTM (Davinch-K Co., Seoul, Korea) (Milne et al., 1993).
7. Statistical analysis
The significance of the experimental results for each compound was assessed using analysis of variance (ANOVA), followed by Duncan's Multiple Range Test (DMRT) at a significance level of 5% (p < 0.05). The results were presented as the means ± standard error (n = 3).
All statistical analyses were conducted using the Statistical Analysis system v.9.1 software (SAS Institute Inc., Cary, NC, USA).
RESULTS AND DISCUSSION
1. Structural determination of sesquiterpene lactones isolated from A. princeps.
Artemprilactone A (1) was isolated as a colorless gum, with a molecular formula of C17H22O5, as determined by 13C nuclear magnetic resonance (NMR) and high resolution electrospray ionization mass spectroscopy (HR ESI-MS) spectroscopic analysis.
In the 1H NMR spectrum of compound 1, three methyl groups (δ 2.08, s, CH3COO–; δ 1.81, s; δ 1.25, d, J = 7.1 ㎐), one exomethylene group (δ 5.22, 1H, s; 5.11, 1H, s), and two oxygenated methane groups (δ 4.85, dt, J = 4.8, 10.4 ㎐; δ 3.98, t, J = 10.4 ㎐) were observed.
The 13C NMR and heteronuclear single quantum coherence (HSQC) spectra revealed the presence of three methyl groups, three methylene groups (including one olefinic carbon), six methine groups (including one olefinic carbon and two oxygenated carbons), and five quaternary carbons (including two olefinic carbons, two carbonyl carbons, and one oxygenated carbon).
The NMR signal pattern indicated that this compound was a sesquiterpene lactone with a guaiane backbone and an acetyl group attached at the C-8 position. The overall structure of this compound was confirmed through 2D NMR experiments, including 1H–1H correlation spectroscopy (COSY), HSQC, and heteronuclear multiple bond correlation (HMBC), leading to its identification as 1-hydroxy-3,10(14)-dien-8-acetyl-guaian-6,12-olide.
A literature search based on this information revealed that the structure of this compound is very similar to that of artemvulactone L, which was previously isolated from A. vulgaris and shares the same structure except for different ester side chains (Chen et al., 2022).
The relative configuration of the asymmetric carbon centers in 1 was determined by the connections observed in nuclear Overhauser effect spectroscopy (NOESY) experiments: H-2α/H-14, H-2α/H-6α, H-5β/H-7β, H-5β/H-9β, H-6α/H-11α, and H-7β/H-13. By analyzing these NOESY correlations, the stereochemistry of compound 1 was determined to be 1S*, 5R*, 6S*, 7R*, 8S*, 11S*. This was confirmed by comparing 13C NMR spectral data of 1 with those of artemvulactone L.
Artemprilactone B (2), a colorless gum, was assigned the molecular formula C17H22O5 based on 13C NMR spectrometry and HRESIMS. The NMR spectral data for 2 were similar to those of 1, with the only difference being the repositioning of the double bond in 1.
In the 1H NMR spectrum, the exomethylene peaks of compound 1 at δ 5.22 (singlet) and δ 5.11 (singlet) disappeared, while a new methyl signal was observed at δ 1.89 with a singlet that did not exhibit any coupling. Additionally, another new olefinic methine peak was detected at δ 5.31, appearing as a doublet of triplets with coupling constants of J = 2.0 and 1.5 ㎐.
Similar changes were found in the 13C NMR spectrum, where the exomethyleneolefinic carbon peak (δ 117.0, CH2) vanished, and one methyl peak (δ 24.4, CH3) and an olefinic methine peak (δ 123.6, CH) were observed. These changes in the signals suggest that 2 was formed by rearrangement of the 10(14)-exomethylene double bond in 1. Through extensive 2D NMR experiments, the position of this double bond was confirmed to be C-9, and the structure of 2 was determined to be 1-hydroxyguai-3,9-dien-6,12-olide 8-O-acetate.
Compound 3 was obtained as a colorless gum, and its molecular formula was determined to be C17H22O6 based on HRESIMS and 13C NMR spectroscopy.
The NMR spectral pattern of 3 was also quite similar to that of 1, but significant differences were observed. In the 1H NMR spectrum, the peak that had appeared at δ 5.50 (1H, s, H-3) in 1 disappeared, and two new peaks were observed at δ 5.88 (1H, d, J = 5.7 ㎐, H-3) and 5.54 (1H, d, J = 5.7 ㎐, H-2).
Corresponding changes were observed in the 13C NMR spectrum. The peak of the 1,1,2-trisubstituted double bond in 1 disappeared; instead, a new peak of a 1,2-disubstituted double bond (δ 141.1, CH; δ 135.4, CH) and one oxygenated quaternary carbon peak (δ 84.0) were observed. All these changes were well explained by shifting the double bond that existed at position C-3 in 1 to position C-2 and introducing a hydroxy group at position C-4. Therefore, the structure of 3 was determined to be 1,4-dihydroxyguai-2,10(14)-dien-6,12-olide 8-O-acetate.
A literature review indicated that this compound corresponded to neoezoguaianin, which was previously isolated from A. montana, and the NMR spectroscopic findings closely matched the values documented in previous studies (Nagaki and Matsueda, 1989).
Compound 4, whose composition was confirmed as C17H22O7 based on 13C NMR and HRESIMS analyses, was isolated as a colorless gum. Although the spectral data of 4 were similar to those of 3, detailed examination of the 1H and 13C NMR data of the two compounds revealed significant differences.
In the 1H NMR spectrum of 4, the four olefinic signals observed at δ 5.88, 5.54, 5.09, and 4.87 in 3 vanished. Instead, two new epoxide methine peaks (δ 3.70, d, J = 1.1 ㎐; δ 3.36, d, J = 1.1 ㎐) and a single methyl peak (δ 1.03, s) emerged.
Similarly, corresponding changes appeared in the 13C NMR spectrum, revealing epoxide oxymethine peaks at δ 58.9 and 58.2 as well as a methyl peak at δ 27.0. These changes aligned well with the structure formed by diepoxidation on both sides of the double bond at C-2 in compound 3. These changes were confirmed by comprehensive 2D NMR experiments. Therefore, the structure of 4 was determined to be 10-hydroxyguaian-6,12-olide-1,2:3,4-diepoxide 8-O-acetate.
A literature survey identified this compound as ezoartemin, which had been isolated from A. montana (Koreeda et al, 1988). However, the assignment of ppm values for some of the carbon atoms in compound 4 was modified based not only on HMBC experimental data but also on NMR spectral data reported for similar compounds (Moyo et al., 2019).
2. Effect of compounds on the viability of HT-1080 cells
The compounds isolated from A. princeps were tested for cytotoxicity to HT-1080 cells using the 3-(4,5-dimethylthiazol-2-yl)-2,5-diphenyl tetrazolium bromide (MTT) assay. HT-1080 cells were exposed to compounds at concentrations from 0 to 10 μM. As depicted in Figure 3, all four compounds showed cell survival rates higher than 80% at concentrations of 10, 5, and 1 μM. Hence, all subsequent experiments were performed below 10 μM, where no toxicity was observed.
3. Effect of compounds on the production of intracellular reactive oxygen species (ROS) in HT-1080 cells
Oxidative modification of various biomolecules, including DNA and proteins, by ROS plays a critical role in the onset of a wide range of diseases, including degenerative diseases. Hence, antioxidants capable of protecting vital molecules crucial for living organisms from oxidative damage may aid in preventing the initiation and advancement of diseases (Juan et al., 2021; Serafini, 2006; Suleman et al., 2019).
2’,7’-dichlorofluorescin diacetate (DCFH-DA) has been extensively used to detect intracellular ROS in biological environments. During the fluorescent labeling process, the nonfluorescent DCFH-DA dye, which readily penetrates the cell, undergoes hydrolysis by intracellular esterases and breaks down into 2’,7’-dichlorodihydrofluororescein (DCFH), which is trapped within the cell. DCFH is then oxidized to 2’,7’-dichlorofluorescein (DCF) by intracellular ROS, emitting fluorescence (Yu et al., 2021; Reiniers et al., 2017).
As shown in Fig. 4, production of ROS was significantly decreased in HT-1080 cells treated with hydrogen peroxide alone (control) and in those pretreated with the compound before exposure to hydrogen peroxide (Fig. 4). Of the compounds tested, compounds 1 and 3 demonstrated particularly strong ROS scavenging effects, showing scavenging rates of 95.8% and 96.5%, respectively, at a concentration of 10 μM for 2 h. Compound 3 suppressed ROS with a scavenging rate of 66.8% even at a concentration of 1 μM.
4. Assessment of the antioxidant capacity of compounds isolated from A. princeps towards genomic DNA
Oxidative DNA damage is triggered by ROS and is believed to play a significant role in mutagenesis, aging, degenerative diseases, and carcinogenesis (Srinivas et al., 2019; Juan et al., 2021). Carcinogenesis is a complex process characterized by mutations in essential genes crucial for cellular homeostasis. Mutations resulting from oxidative DNA damage are suspected to be major contributors to cancer (Klaunig and Wang, 2018).
Approximately 80% of DNA damage associated with cancer development is caused by ROS, such as hydrogen peroxide (H2O2), singlet oxygen (1O2), and hydroxyl radicals (.OH) (Wiseman and Halliwell, 1996). Among these, hydroxyl radicals are the most reactive oxygen radicals generated within organisms via the Fenton reaction. Traditionally perceived as a detrimental byproduct of oxidative metabolism, these radicals have the capacity to inflict molecular damage within the body while also serving as a pivotal catalyst for various radical reactions in the presence of oxygen (Juan et al., 2021; Gligorovski et al., 2015).
In the present study, the protective effect of guaianolides (1–4) against DNA oxidative damage caused by hydroxyl radicals generated through the Fenton reaction was evaluated by observing the expression levels of genomic DNA from HT-1080 cells by electrophoresis. The expression level of DNA untreated with both sample and hydroxyl radicals generated in the Fenton reaction was set to 100% (blank), and the relative reducing power of each sample was expressed as a percentage relative to the blank.
These were then compared with the treatment group (control), which only included the hydroxyl radicals generated by the Fenton reaction. All compounds exhibited significant protective effects against DNA oxidative damage at a concentration of 10 μM, with values of 59.5%, 58.3%, 50.0%, and 90.5%, respectively. Additionally, at a concentration of 1 μM, the compounds still showed over 50% protection (Fig. 5).
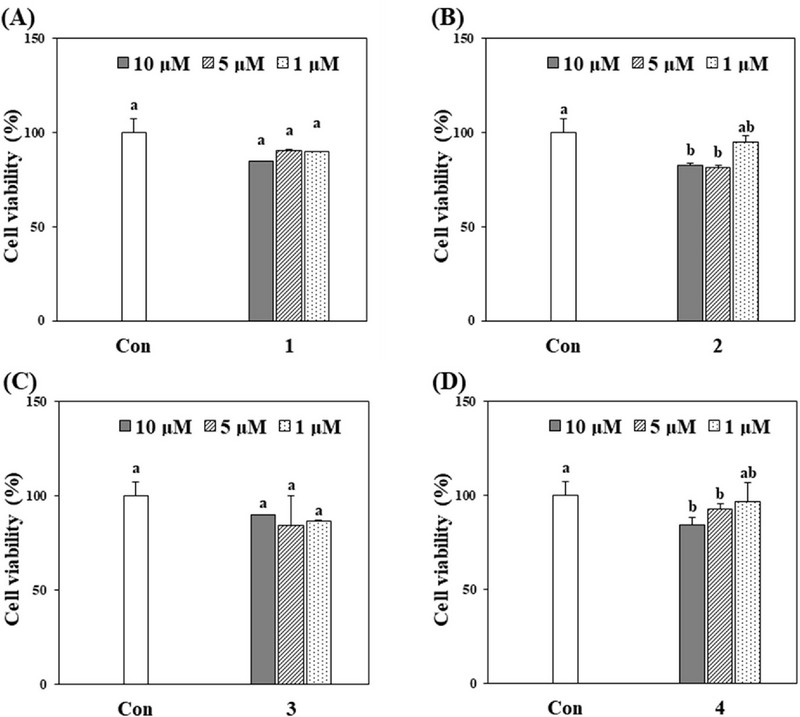
Effect of compounds (1–4) on the viability of HT-1080 cells.The evaluation of all four compounds revealed no considerable cytotoxic effects on HT-1080 cells. The survival rate of HT-1080 cells was calculated as a percentage relative to untreated cells. Results are expressed as the means ± standard deviation (SD) from three separate experiments (n = 3). The absence of statistically significant differences is indicated by identical letters ( p > 0.05).
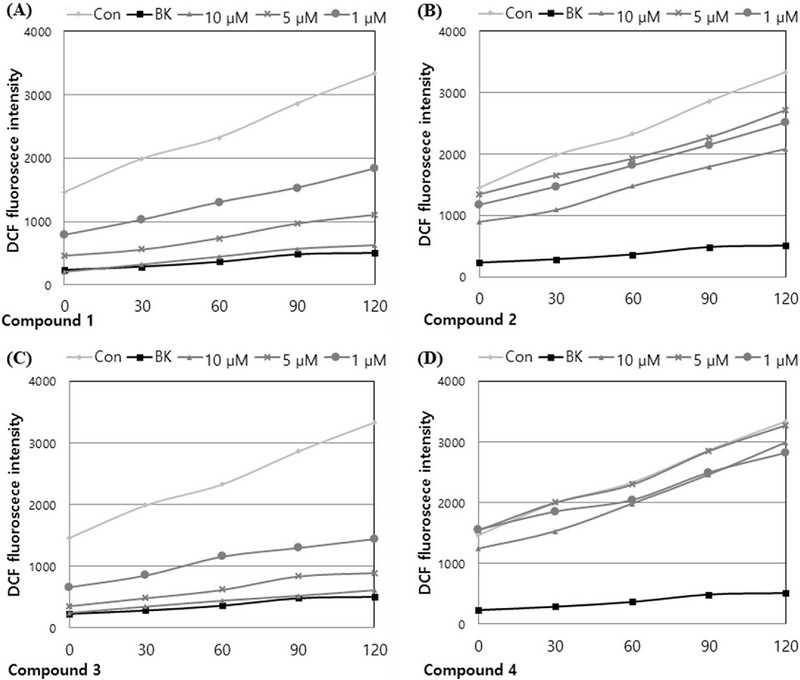
Effects of compounds 1–4 (A–D) from A. princeps on intracellular ROS levels induced by hydrogen peroxide in HT-1080 cells.
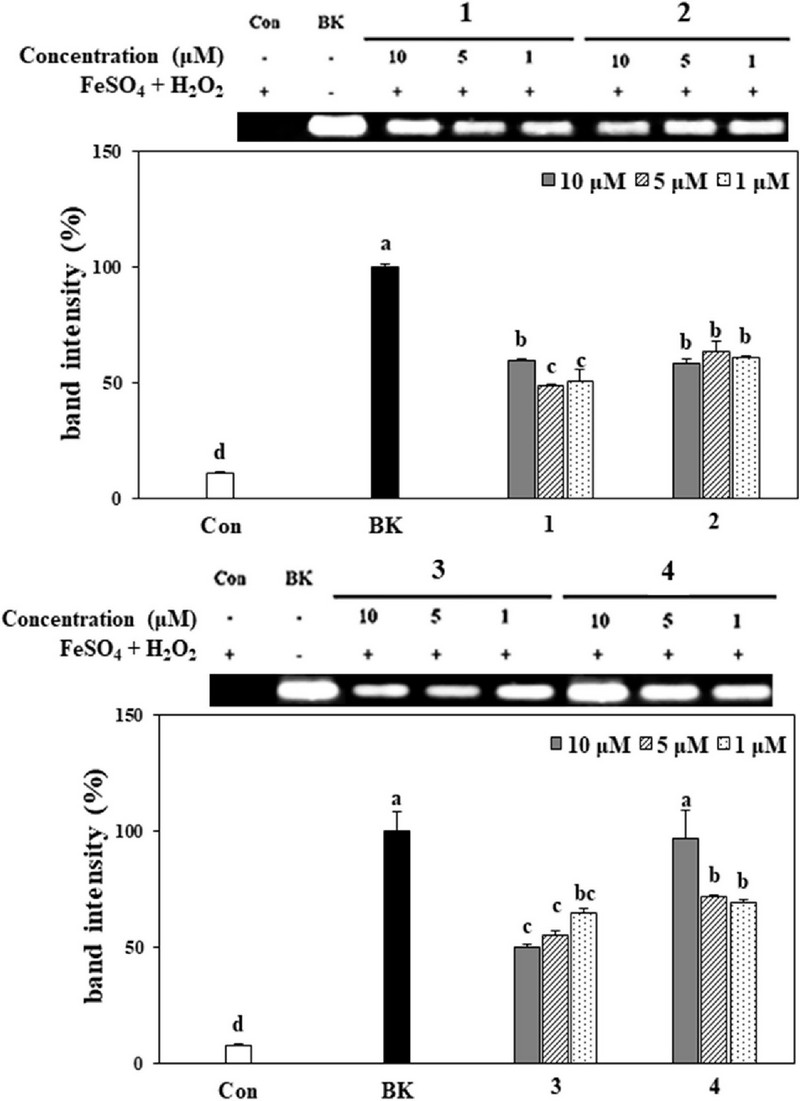
Protective effect of compounds 1–4 from A. princeps on genomic DNA in HT-1080 cells.Band sizes were calculated and depicted as percentage compared to the blank group. Values are the means ± SD. *Means with different letters (a - d) at the same concentration are significantly different at 5% by Duncan’s Multiple Range Test (DMRT, p < 0.05).
Guaianolides, a class of sesquiterpenes, exhibit diverse biological activities, including antitumor, anti-inflammatory, and antibacterial effects. These sesquiterpene lactones are not only abundant in number but also have complex and diverse structures.
They have been isolated from a variety of biological sources, including marine animals, but most of them are mainly found in the Asteraceae and Apiaceae families, which are terrestrial plants (Fraga, 2007; Simonsen et al., 2013; Asem and Laitonjam, 2014; Rehman et al., 2016; Ma et al., 2019; Mehrabani et al., 2023).
In this study, two new guian-6,12-olides (1 and 2) and two known guian-6,12-olides (3 and 4) were isolated from Artemisia princeps of the Asteraceae family. Although the biological activities of guian-6,12-olides are known (Simonsen et al., 2013; Ma et al., 2019), little is known about the antioxidant activity of guian-6,12-olides (Asem and Laitonjam, 2014; Rehman et al., 2016; Mehrabani et al., 2023).
The antioxidant activity of the isolated guaia-6,12-olides was evaluated using intracellular ROS-scavenging and genomic DNA cleavage assays. Intracellular ROS levels were significantly inhibited by compounds 1 and 3. Additionally, all compounds showed protective effects against oxidative DNA damage caused by hydroxyl radicals, which can lead to cancer.
In conclusion, our data suggest that guaian-6,12-olide can be used as a lead compound for the development of novel antioxidants. However, additional research is needed on the mechanism by which these types of guaianolides act as antioxidants.
Acknowledgments
This research was funded by the Basic Science Research Program of the National Research Foundation of Korea(NRF), under the auspices of the Ministry of Science and ICT(No. 2022R1F1A1065328).
References
-
Asem SD and Laitonjam WS. (2014) A new guaianolidesesquiterpene lactone from Curcuma leucorrhiza Roxb. Natural Product Research. 28:477-482.
[https://doi.org/10.1080/14786419.2013.879306]
- Bang MH, Song MC, Han MW, Lee DY, Jo JK, Chung HG, Jeong TS, Lee KT, Choi MS and Baek NI. Development of biologically active compounds from edible plant sources-XIX. Isolation of inhibitory compound on LDL-oxidation from the aerial parts of Sajabalssuk(Artemisia princeps Pampanini, Sajabalssuk). (2007). Journal of the Korean Society for Applied Biological Chemistry.50:224-227.
-
Chen XY, Liu T, Hu YZ, Qiao TT, Wu XJ, Sun PH, Qian CW, Ren Z, Zheng JX and Wang YF. (2022). Sesquiterpene lactones from Artemisia vulgaris L. as potential NO inhibitors in LPS-induced RAW264.7 macrophage cells. Fronters in Chemistry. 10:948714. https://www.frontiersin.org/journals/chemistry/articles/10.3389/fchem.2022.948714/full, (cited by 2024 June 8).
[https://doi.org/10.3389/fchem.2022.948714]
-
Dhyani P, Sati P, Sharma E, Attri DC, Bahukhandi A, Tynybekov B, Szopa A, Sharifi-Rad J, Calina D, Suleria HAR and Cho WC. (2022). Sesquiterpenoid lactones as potential anti-cancer agents: an update on molecular mechanisms and recent studies. Cancer Cell International. 22:305. https://link.springer.com/article/10.1186/s12935-022-02721-9, (cited by 2024 June 8).
[https://doi.org/10.1186/s12935-022-02721-9]
-
Drew DP, Krichau N, Reichwald K and Simonsen HT. (2009). Guaianolides in Apiaceae: perspectives on pharmacology and biosynthesis. Phytochemistry Reviews. 8:581-599.
[https://doi.org/10.1007/s11101-009-9130-z]
-
Fraga BM. (2007). Natural sesquiterpenoids. Natural Product Reports. 24:1350-1381.
[https://doi.org/10.1039/b706427f]
-
Gligorovski S, Strekowski R, Barbati S and Vione D. (2015). Environmental implications of hydroxyl radicals(?OH). Chemical Reviews. 115:13051-13092.
[https://doi.org/10.1021/cr500310b]
-
Juan CA, Pérez de la Lastra, JMP, Plou FJ and Pérez-Lebeña E. (2021). The chemistry of reactive oxygen species(ROS) revisited: outlining their role in biological macromolecules (DNA, lipids and proteins) and induced pathologies. International Journal of Molecular Sciences. 22:4642. https://www.mdpi.com/1422-0067/22/9/4642, (cited by 2024 June 8).
[https://doi.org/10.3390/ijms22094642]
- Karadeniz F, Oh JH and Kong CS. (2021). Sesquiterpene lactones: A review of biological activities. Journal of Life Science. 31:430-441.
-
Klaunig JE and Wang Z. (2018). Oxidative stress in carcinogenesis. Current Opinion in Toxicology. 7:116-121.
[https://doi.org/10.1016/j.cotox.2017.11.014]
-
Koreeda M, Nagaki M, Hayami KI and Matsueda S. (1988). Studies on sesquiterpene lactones. XIII. : Chemical constituents of Artemisia montana (NAKAI) PAMP.Yakugaku Zasshi. 108:434-436.
[https://doi.org/10.1248/yakushi1947.108.5_434]
- Li D, Han XH, Hong SS, Lee C, Lee MS, Lee D, Lee MK and Hwang BY. Inhibitors of nitric oxide production from Artemisia princeps. (2010). Natural Product Sciences. 16:143-147.
-
Ma GH, Chen, KX, Zhang LQ and Li YM. (2019). Advance in biological activities of natural guaiane-type sesquiterpenes. Medicinal Chemistry Research. 28:1339-1358.
[https://doi.org/10.1007/s00044-019-02385-7]
-
Mehrabani M, Iranshahi M, Mehrabani M, Jafari M, Zadeh MA and Farhadi F. (2023). Evaluation of cytotoxic and antioxidant effect of guaianolid sesquiterpenes from Ferula hindukushensis. ChemistrySelect. 8:e202300895. https://chemistry-europe.onlinelibrary.wiley.com/doi/abs/10.1002/slct.202300895, (cited by 2024 June 8).
[https://doi.org/10.1002/slct.202300895]
-
Milne L, Nicotera P, Orrenius S and Burkitt MJ. (1993). Effects of glutathione and chelating agents on copper-mediated DNA oxidation: pro-oxidant and antioxidant properties of glutathione. Archives of Biochemistry and Biophysics. 304:102-109.
[https://doi.org/10.1006/abbi.1993.1327]
-
Mosmann T. (1983). Rapid colorimetric assay for cellular growth and survival: application to proliferation and cytotoxicity assays. Journal of Immunological Methods. 65:55-63.
[https://doi.org/10.1016/0022-1759(83)90303-4]
-
Moujir L, Callies O, Sousa PMC, Sharopov F and Seca AML. (2020). Applications of sesquiterpene lactones: A review of some potential success cases. Applied Sciences. 10:3001. https://www.mdpi.com/2076-3417/10/9/3001, (cited by 2024 June 8).
[https://doi.org/10.3390/app10093001]
-
Moyo P, Kunyane P, Selepe MA. Eloff JN, Niemand J, Louw AI, Maharaj VJ and Birkholtz LM. (2019). Bioassay-guided isolation and identification of gametocytocidal compounds from Artemisia afra(Asteraceae). Malaria Journal. 18:65. https://link.springer.com/article/10.1186/s12936-019-2694-1, (cited by 2024 June 8).
[https://doi.org/10.1186/s12936-019-2694-1]
-
Nagaki M, Matsueda S. (1989). Guaianolides from Artemisia montana. Phytochemistry. 28:2731-2733.
[https://doi.org/10.1016/S0031-9422(00)98077-4]
-
Nawrot J, Adamski Z, Kamińska-Kolat B, Kubisiak-Rzepczyk H, Kroma A, Nowak G and Gornowicz-Porowska J. (2021). Antifungal activity of the sesquiterpene lactones from Psephellus bellus. Plants. 10:1180. https://www.mdpi.com/2223-7747/10/6/1180, (cited by 2024 June 8).
[https://doi.org/10.3390/plants10061180]
-
Okimotoa Y, Watanabea A, Nikia E, Yamashitab T and Noguchia N. (2000). A novel fluorescent probe diphenyl-1-pyrenylphosphine to follow lipid peroxidation in cell membranes. FEBS Letters. 474:137-140.
[https://doi.org/10.1016/S0014-5793(00)01587-8]
-
Rehman NU, Hussain H, Al-Riyami SA, Csuk R, Khiat M, Abbas G, Al-Rawahi A, Green IR, Ahmed I and Al-Harrasi A. (2016). Lyciumaside and lyciumate: A new diacylglycoside and sesquiterpene lactone from Lycium shawii. Helvetica Chimica Acta. 99:632-635.
[https://doi.org/10.1002/hlca.201600066]
-
Reiniers MJ, van Golen RF, Bonnet S, Broekgaarden M, van Gulik TM, Egmond MR and Heger M. (2017). Preparation and practical applications of 2’,7’-dichlorodihydrofluorescein in redox assays. Analytical Chemistry. 89:3853-3857.
[https://doi.org/10.1021/acs.analchem.7b00043]
-
Salazar-Gómez A, Ontiveros-Rodríguez, JC, Pablo-Pérez SS, Vargas-Díaz ME and Garduño-Siciliano L. (2020). The potential role of sesquiterpene lactones isolated from medicinal plants in the treatment of the metabolic syndrome — A review. South African Journal of Botany. 135:240-251.
[https://doi.org/10.1016/j.sajb.2020.08.020]
-
Serafini M. (2006). The role of antioxidants in disease prevention. Medicine. 34:533-535.
[https://doi.org/10.1053/j.mpmed.2006.09.007]
-
Simonsen HT, Weitzel C and Christensen SB. (2013). Guaianolide sesquiterpenoids: pharmacology and biosynthesis. In Ramawat KG and Mérillon JM. (eds.). Natural Products: Phytochemistry, botany and metabolism of alkaloids, phenolics and terpenes, Springer Berlin Heidelberg. Berlin, Germany. p.3069-3097.
[https://doi.org/10.1007/978-3-642-22144-6_134]
-
Srinivas US, Tan BWQ, Vellayappan BA and Jeyasekharan AD. (2019). ROS and the DNA damage response in cancer. Redox Biology. 25:101084. https://www.sciencedirect.com/science/article/pii/22S13231718309017, (cited by 2024 June 8).
[https://doi.org/10.1016/j.redox.2018.101084]
- Suleman M, Khan A, Baqi A, Kakar MS, Samiullah and Ayub M. (2019). Antioxidants, its role in preventing free radicals and infectious diseases in human body. Pure and Applied Biology. 8:380-388.
-
Vajs V, Todorović N, Ristić M, Tesević V, Todorović B, Janaćković P, Marin P and Milosavljević S. (1999). Guaianolides from Centaureanicolai: Antifungal activity. Phytochemistry. 52:383-386.
[https://doi.org/10.1016/S0031-9422(99)00207-1]
-
Wedge DE, Galindo JCG and Macías FA. (2000). Fungicidal activity of natural and synthetic sesquiterpene lactone analogs. Phytochemistry. 53:747-757.
[https://doi.org/10.1016/S0031-9422(00)00008-X]
-
Wiseman H and Halliwell B. (1996). Damage to DNA by reactive oxygen and nitrogen species: role in inflammatory disease and progression to cancer. Biochemical Journal. 313:17-29.
[https://doi.org/10.1042/bj3130017]
-
Yu D, Zha Y, Zhong Z, Ruan Y, Li Z, Sun L and Hou S. (2021). Improved detection of reactive oxygen species by DCFH-DA: New insight into self-amplification of fluorescence signal by light irradiation. Sensors and Actuators B: Chemical. 339:129878. https://www.sciencedirect.com/science/article/abs/pii/S0925400521004470, (cited by 2024 June 8).
[https://doi.org/10.1016/j.snb.2021.129878]